This article covers
What is Cell Culture? A Quick Overview
Cell culture is a technique used in the laboratory to grow and maintain cells in an artificial environment, typically in a Petri dish or flask.
Depending on the sources of cells, cell culture can be divided into the cultivation of primary cells (cells obtained directly from the original tissues or organs) or cell lines (established cell strains with unlimited life spans).
[In this video] See cells in motion under a live microscope.
Cell culture allows scientists to study the growth, development, and other characteristics of cells under controlled conditions.
Cell culture is an important tool in many areas of research, including molecular biology, biochemistry, genetics, and pharmacology.
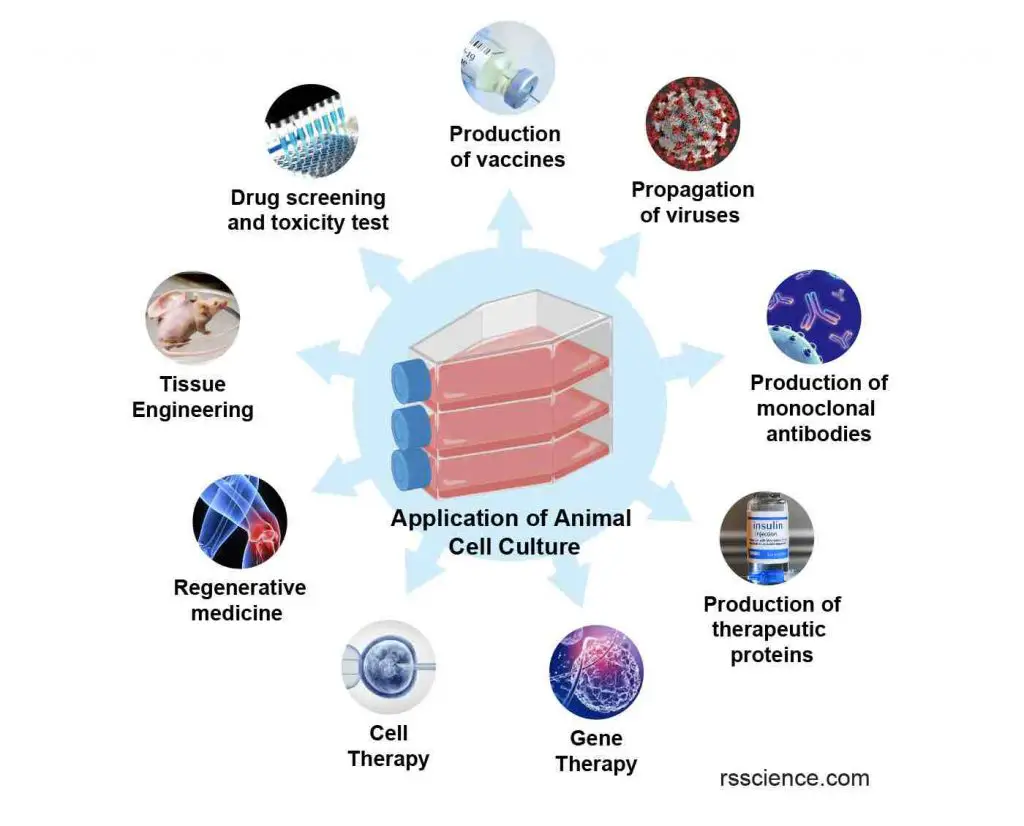
[In this image] Various applications of cell culture techniques in basic and medical research, drug development, vaccine and therapeutic protein production, gene and cell therapy, and regenerative medicine. See “Applications of Cell Culture” for detail.
What is “Cell”?
Cells are building blocks of our bodies. They are the smallest unit of an organism that is capable of performing all the functions necessary for life.
You can think of a cell as a balloon filled with a nutrient-rich solution. The thin, flexible film that surrounds the cell and separates the inside from the outside environment is called the cell membrane.
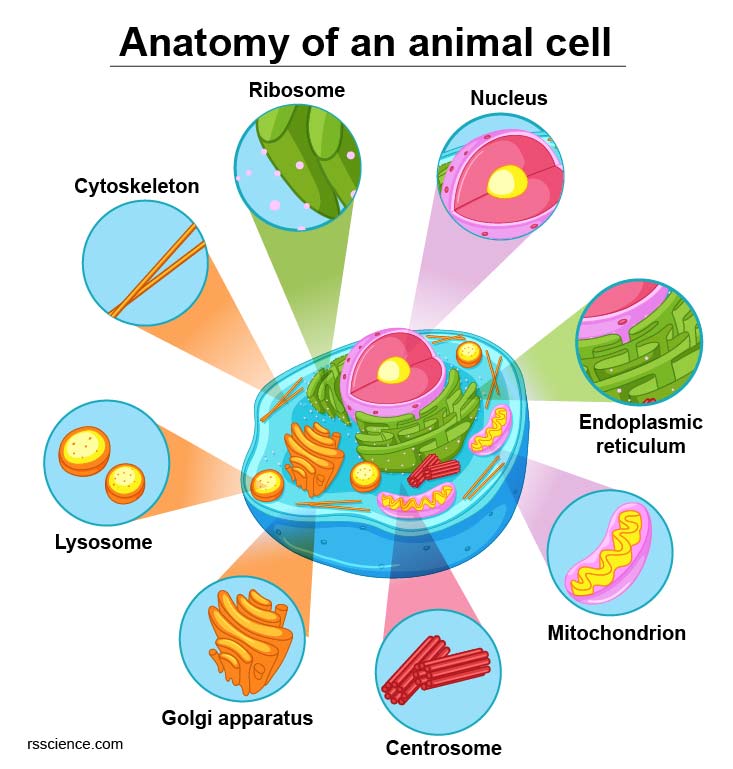
[In this figure] Diagram of an animal cell including several types of cell organelles.
Extended reading: Animal vs. Plant cells – Similarities, Differences, Chart, and Examples
Inside the cell membrane are various cell organelles, which are tiny cellular structures that perform specific functions within the cell. These organelles can be thought of as the internal organs of the cell. For example, the nucleus serves as the cell’s “brain,” while the mitochondria act as the cell’s “heart.”
Extended reading: Cell Organelles and their Functions
The human body is composed of over 200 different types of cells, including white blood cells, red blood cells, nerve cells, heart muscle cells, and epithelial cells. Each type of cell has a specific role in the body, and all cells work together to maintain the overall health and proper function of the organism.
Table. Common Primary Cell Types
Epithelial | Endothelial | Fibroblasts | Keratinocytes | Melanocytes | Neurons |
Astrocytes | Hepatocytes | Skeletal Muscle | Smooth Muscle | Osteoblasts | Myocytes |
Chondrocytes | Adipocytes | Synoviocytes | Hair Cells | Blood Cells | Stem Cells |
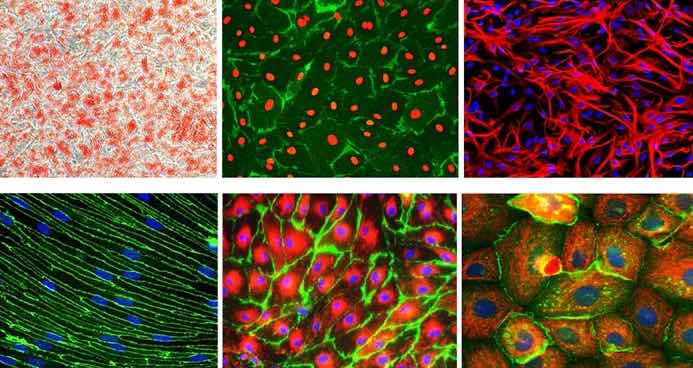
[In this image] Immunofluorescent images of several commonly used primary cells in biomedical research.
Image source: Sigma
It is estimated that the human body contains about 37.2 trillion cells.
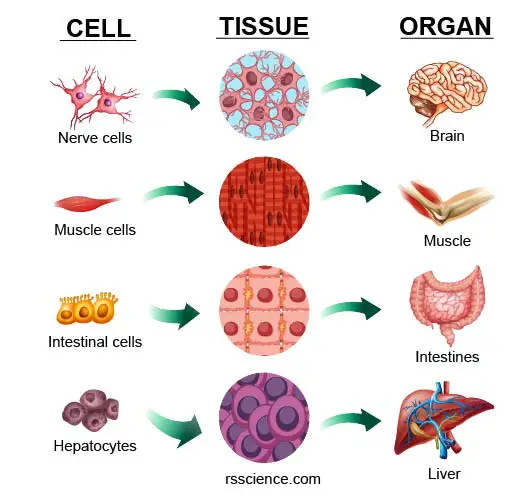
[In this image] Many cells assemble into a specific type of “tissue.” One or more tissues work together as an “organ.”
Why do we need “Cell Culture”?
Observing human cells is crucial for understanding human health and treating diseases. By studying cells, we can gain insight into how they function and use this knowledge to diagnose, monitor, and treat various conditions. To effectively study cells, it is necessary to have a way to observe them continuously.
Ideally, we should be able to “pet” cells – growing them in a fish tank so that we can observe them closely. This technique is called “Cell Culture”.
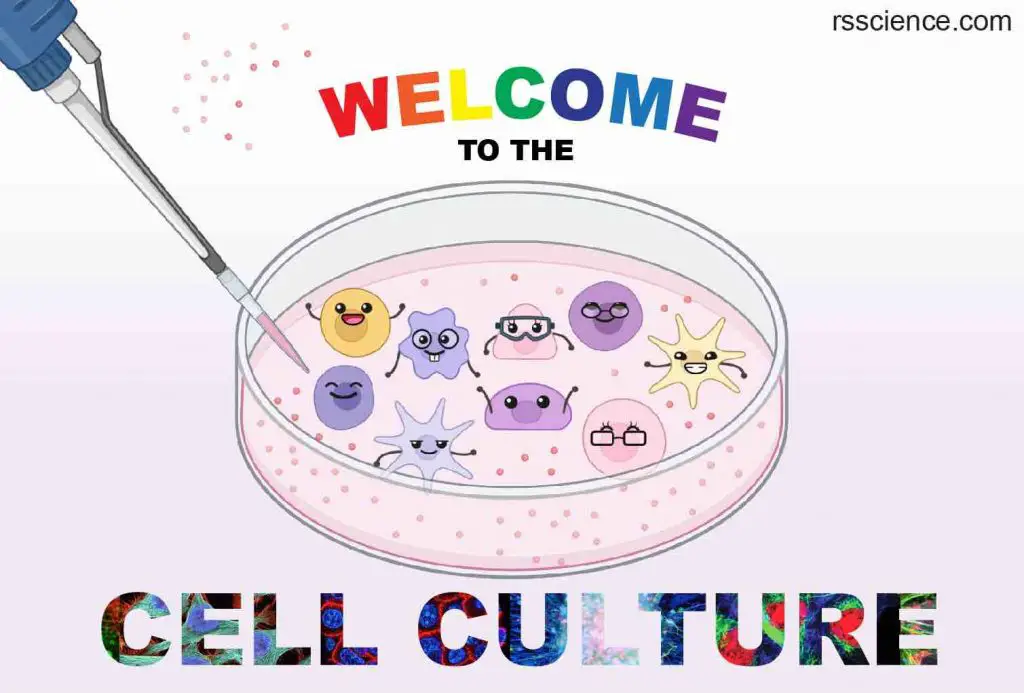
Produced by Biorender.com
Cell culture is a technique that involves growing cells in a laboratory setting, such as in a culture dish or flask. These cell culture containers are transparent to allow us closely observing cells under a microscope.
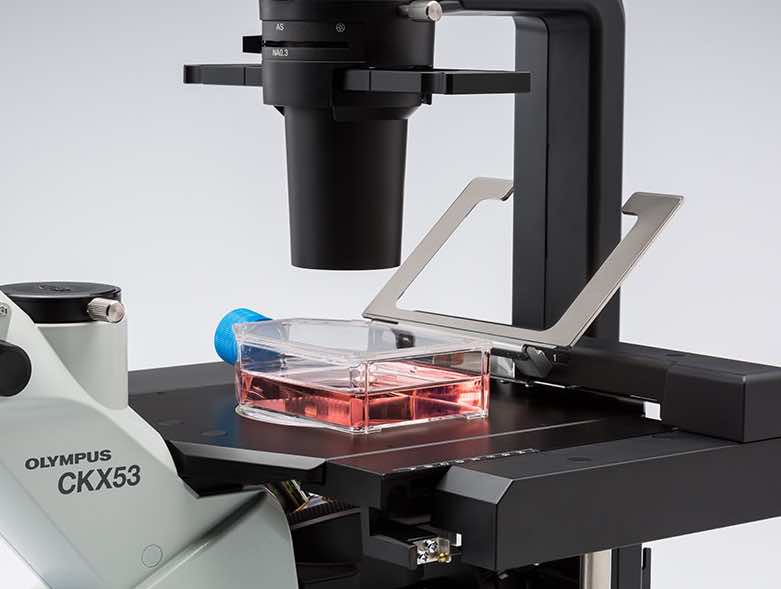
[In this image] The cell culture vessels or containers are made of transparent plastics. You can see the cultured cells through the container walls under an inverted microscope.
Image source: Olympus
Like taking care of your pet fish, scientists can tell whether their cultured cells are happy or not. For example, by providing the cells with different nutrients, researchers can learn more about the body’s energy metabolism. By introducing unknown chemicals or toxins to the cells, researchers can study toxicology and understand how certain substances affect cell health.
Additionally, by manipulating the genes of the cells, researchers can learn more about the functions of specific genes. All of these studies rely on the ability to grow human cells in the laboratory, which is what we refer to as cell culture. This technique is critical for understanding how cells work and is an essential tool in the study of human health and the treatment of diseases.
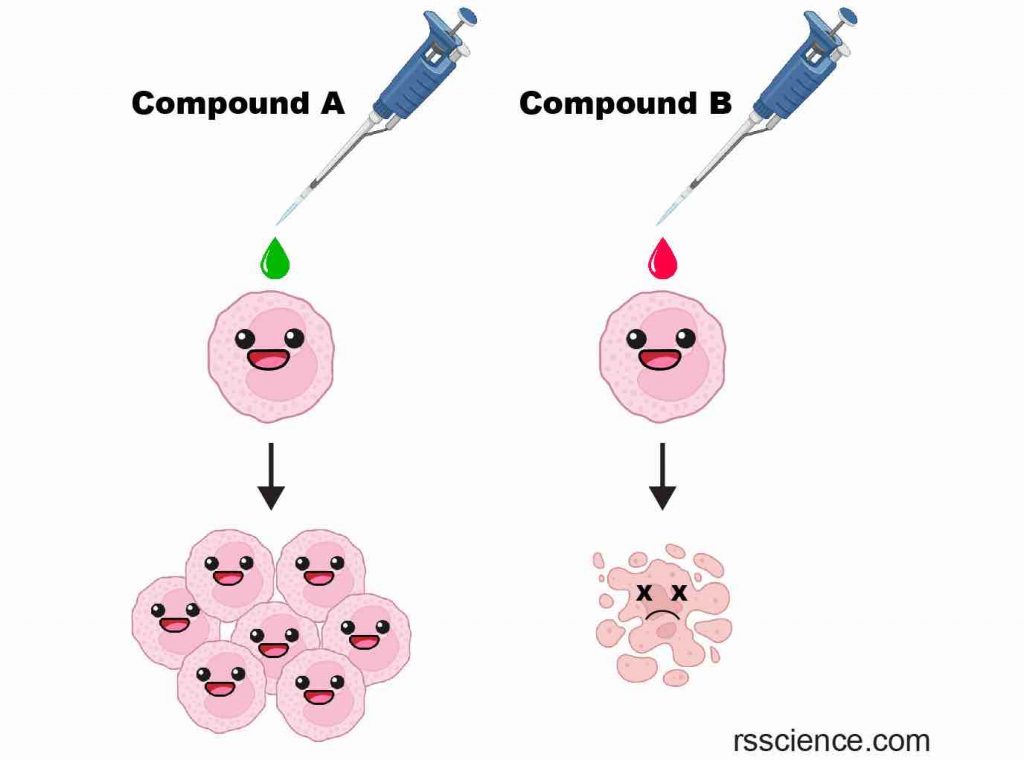
[In this image] Examples of testing chemicals on cultured cells.
By adding Compound A, cells grow much faster, suggesting Compound A is beneficial to the cells. On the other hand, Compound B kills the cells, suggesting Compound B is toxic.
How to Culture Cells? – The Principle of Cell Culture
To keep your pet fish happy, it is important to replicate their natural habitat as closely as possible. The same principle applies to cell culture. Scientists have spent decades researching the ideal conditions for keeping cells happy in culture dishes. Here are some key factors to consider:
- A nutrient-rich medium
- A flat surface for cells to anchor (except for blood cells)
- A neutral pH (pH 6.8 ~ 7.4)
- A body temperature (37oC or 98.6oF)
- An isotonic osmotic pressure
- A source of oxygen
- Regular removal of wastes
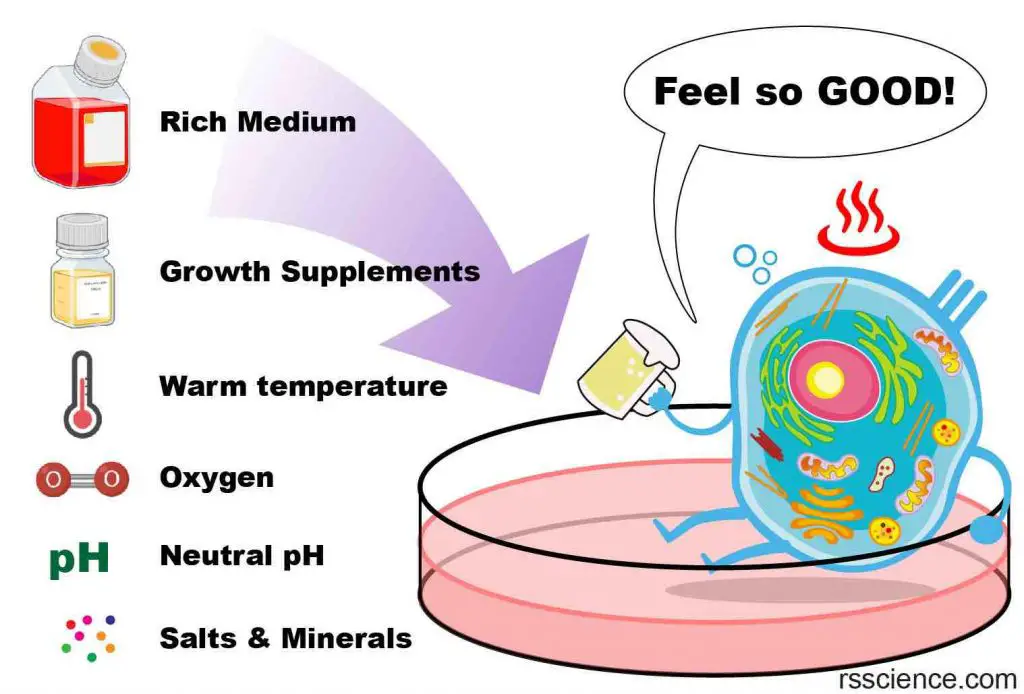
Table. Optimal cell culture conditions for most mammalian cells.
pH | 7.0–7.4 |
Osmolarity | 280–320 mOsmol/kg |
CO2 | 5–10% |
Temperature | 35–37 oC |
Cell Culture Medium
Cells are grown in a nutrient-rich medium that provides them with the necessary nutrients, growth factors, hormones, and gases (O2) to thrive. This medium also provides a stable physical and chemical environment with a suitable pH, osmotic pressure, and temperature.
Scientists try to replicate the conditions of our body fluids in the cell culture medium, with a neutral pH (pH 6.8 ~ 7.4), body temperature (37oC or 98.6oF), and isotonic osmotic pressure. In addition, necessary nutrients such as amino acids, vitamins, inorganic salts, and a carbon source like glucose are added to the medium according to the cells’ physiological needs. These formulations are known as basal media.
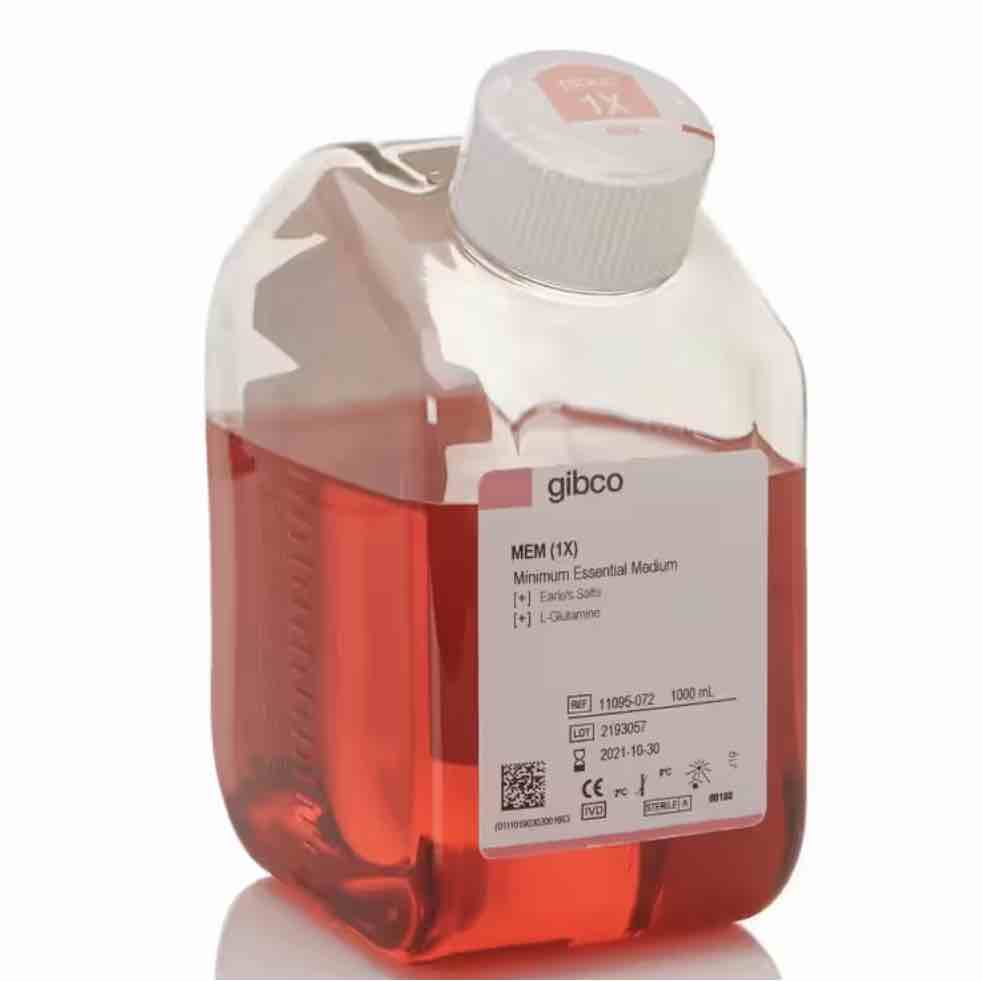
[In this image] A bottle of commercial Minimum Essential Medium (MEM).
Other common basal media include Dulbecco’s Modified Eagle Medium (DMEM), RPMI1640, and Basal Medium Eagle (BME).
Image source: ThermoFisher
Serum is a component of blood that contains many proteins, hormones, enzymes, and growth factors that support the cells in our body. It is common to add a percentage (5-20%) of fetal bovine serum (FBS) to the cell culture medium as a supplement. Some cell types may require additional growth factors, such as neural growth factors (NGF) for the growth of neural cells.
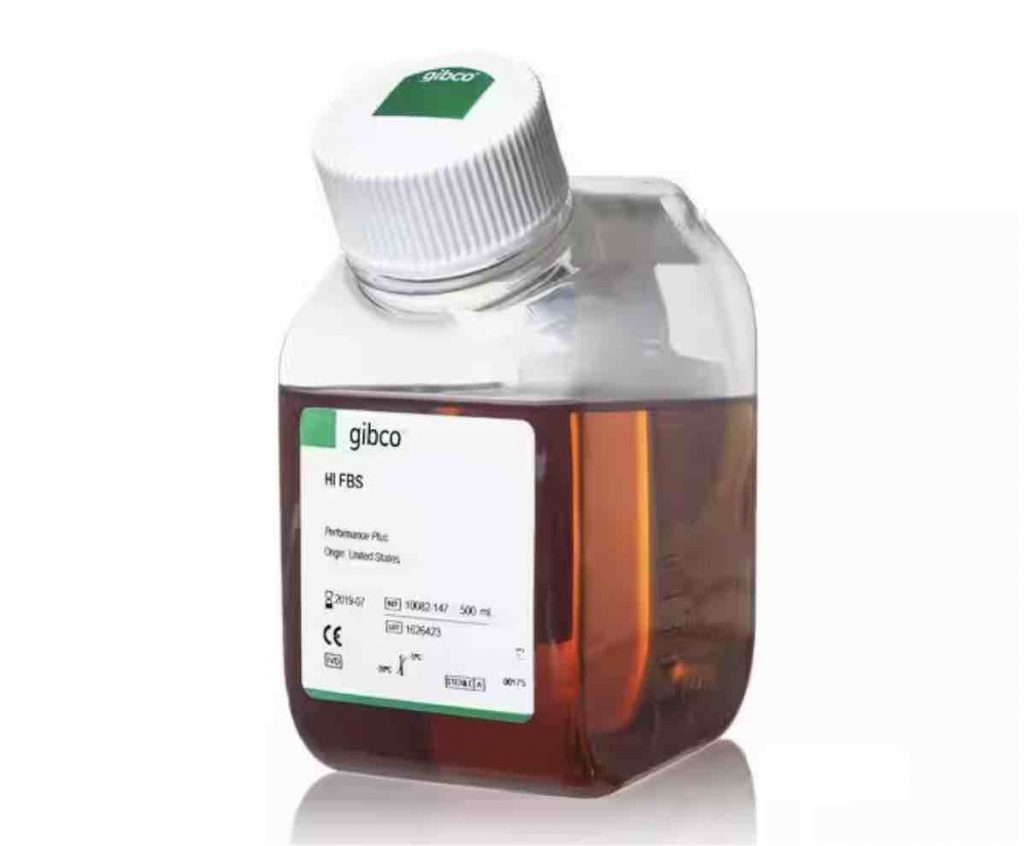
[In this image] A bottle of commercial fetal bovine serum.
Image source: ThermoFisher
Cell culture medium is often supplemented with an antibiotic combination of penicillin and streptomycin as a measure to prevent bacterial growth.
Carbon dioxide (CO2)
The growth medium controls the pH of the cell culture and helps to buffer the cells against changes in pH. This is typically done by including an organic buffer, like HEPES, or a CO2-bicarbonate buffer. The pH of the medium is dependent on the balance of dissolved CO2 and bicarbonate, and changes in atmospheric CO2 can alter the pH of the medium. To prevent this, it is necessary to use exogenous CO2 when using a CO2-bicarbonate buffer. Most researchers use a concentration of 5% CO2.
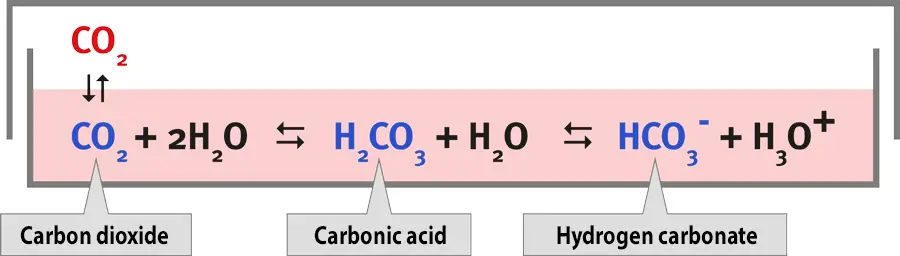
[In this image] The pH values in media depend on the concentration of CO2 in the incubator.
Image source: Binder GmbH
Cell Culture Containers – Dishes or Flasks
Anchorage-dependent cells require a solid or semi-solid substrate to form an adherent culture as a monolayer. Researchers typically use plastic cell culture containers, such as dishes, flasks, and multi-well plates, to grow these cells. These containers are chosen for different experimental purposes.
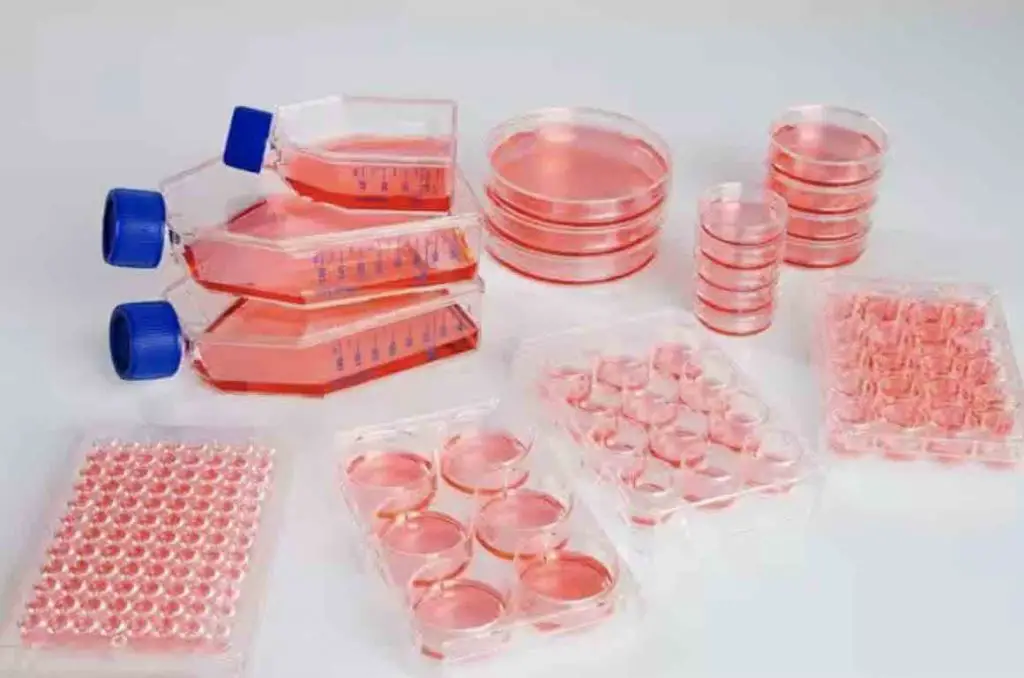
[In this image] Cell culture flasks, dishes, and multi-well plates.
Image source: ThermoFisher
Blood cells can be grown floating in the culture medium as a suspension culture. Small-scale suspension cultures can be done in cell culture flasks or plates. Large-scale suspension cultures are typically done in special spinner flasks (i.e., stirrer bottles), which are designed for suspension cell culture. These flasks allow for better gas exchange and can accommodate larger volumes of cells.
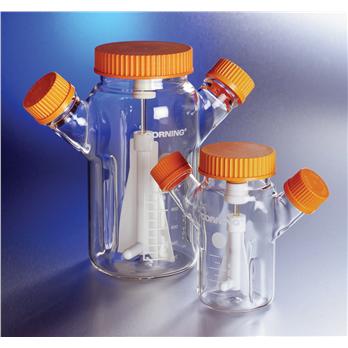
[In this image] Glass spinner flasks for suspension culture.
Image source: Thomas Scientific
Cell Culture Incubator
The cell culture incubator is designed to create the optimal environment for cell growth. Typical incubators have forced humid air circulation and temperature control. These incubators are connected to a CO2 cylinder with a regulator to maintain a desired concentration of CO2 (usually 5%).
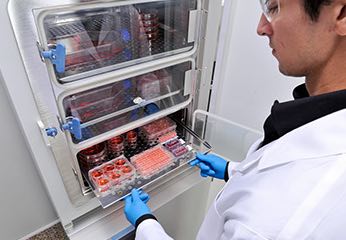
[In this image] Cells are grown in flasks, dishes, and plates that are placed inside an incubator with strictly controlled humidity, temperature, and CO2 concentration. This helps to provide the optimal environment for cell growth and proliferation.
Image source: ThermoFisher
Aseptic Technique
Performing housekeeping for cultured cells involves replenishing the medium and removing waste. To do so, we must operate every step very carefully, through a process known as the aseptic technique.
Successful cell culture depends heavily on the practice of an aseptic technique to keep the cells free from contamination by microorganisms such as bacteria, fungi, and viruses. The elements of the aseptic technique include a sterile work area, good personal hygiene, sterile reagents and media, and sterile handling.
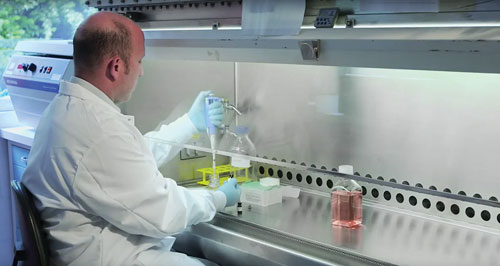
[In this image] A researcher is working with cell culture in a cell culture hood.
Image source: Genetic Engineering & Biotechnology News
Sterile handling of cell culture is usually done inside a cell culture hood (also known as laminar flow hood). The hood consists of a work surface enclosed in a cabinet with a front opening, through which the user can access the work area. A laminar flow of air is generated within the hood, which helps to remove contaminants from the air and maintain the sterility of the work area.
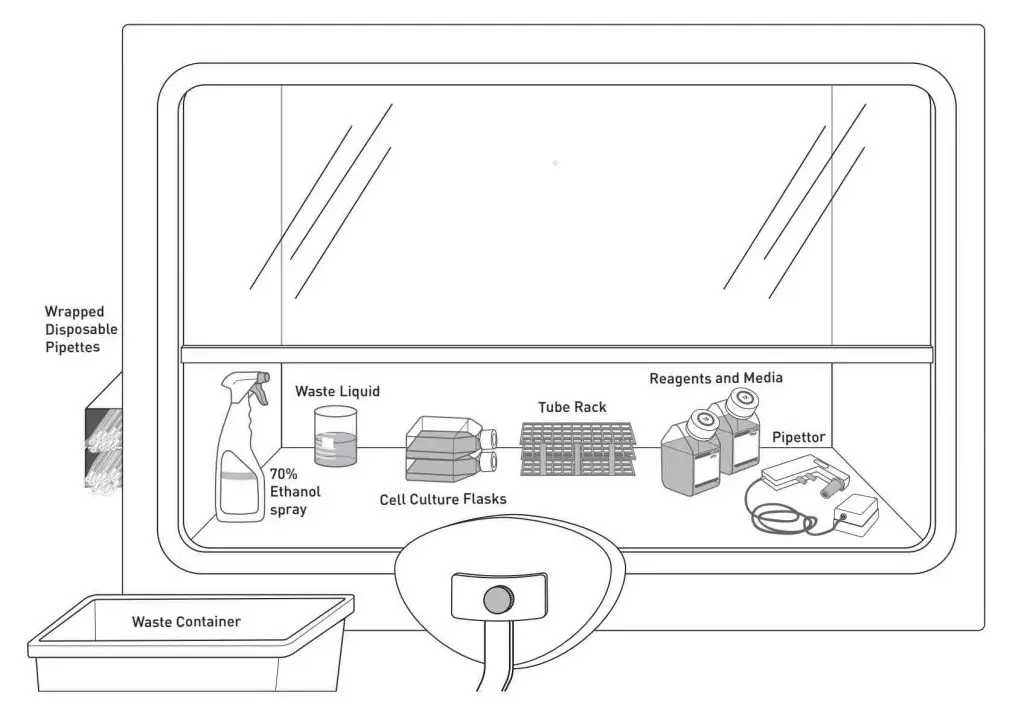
[In this image] The basic layout of a cell culture hood.
Image source: Invitrogen
Cell Culture Laboratory
Most laboratories have dedicated cell culture rooms. This is good not only for preventing the contamination of cell cultures, but also to eliminate exposure of laboratory workers and the outside environment to potentially harmful biological agents.
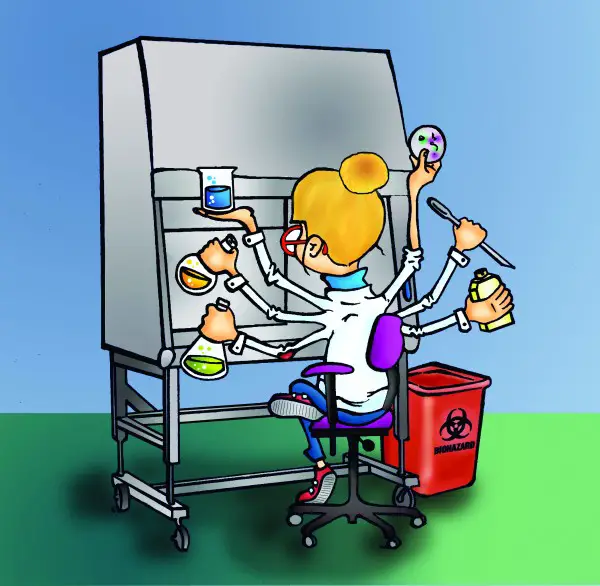
Image credit: Caroline Norman
The Centers for Disease Control (CDC) in the United States sets regulations and recommendations for biosafety in laboratory settings. Cell culture laboratories can be assigned to one of four biosafety levels based on the risk associated with handling a particular agent.
Biosafety Level 1 (BSL-1) is the basic level of protection and is appropriate for agents that do not cause disease in normal, healthy humans.
Biosafety Level 2 (BSL-2) is appropriate for moderate-risk agents that can cause human disease through ingestion, inhalation, or injection. Most cell culture labs should be at least BSL-2.
Biosafety Level 3 (BSL-3) is appropriate for agents that have the potential for aerosol transmission and may cause serious and potentially lethal infections.
Biosafety Level 4 (BSL-4) is the highest level of containment and is appropriate for agents that pose a high individual risk of life-threatening disease through infectious aerosols and for which no treatment is available. These agents are restricted to high-containment laboratories.
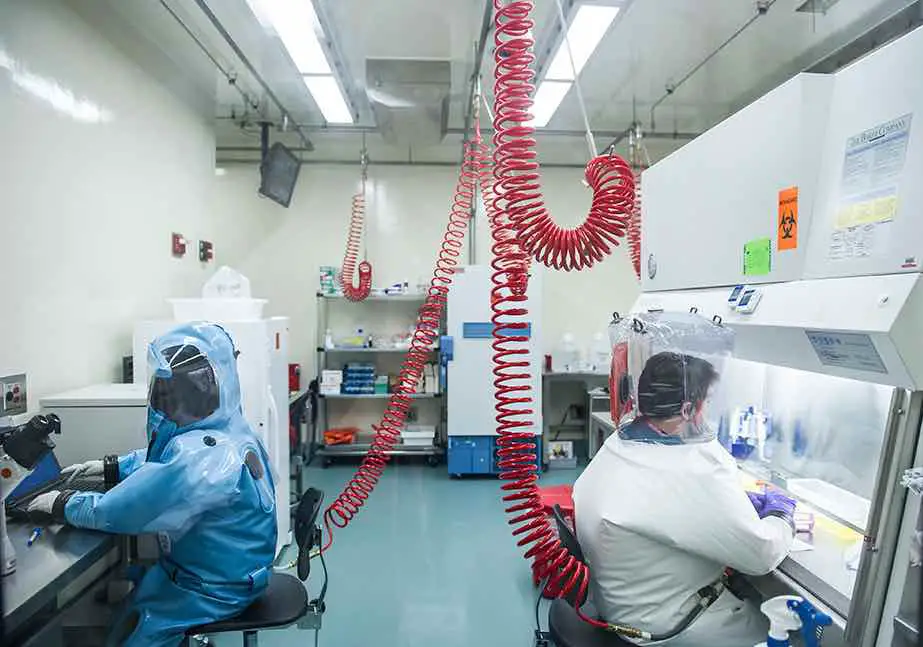
[In this image] In a BSL-4 cell culture room of Boston University, scientists are studying a Level-4 pathogen, the Ebola virus.
Image source: Boston University
Passaging cells
Healthy cells proliferate in the cell culture by cell division. However, once the cells have filled all available space on the substrate (reached confluence), it is necessary to transfer them to a new dish with a fresh growth medium to provide more room for growth. This process is known as subculturing or passaging cells.
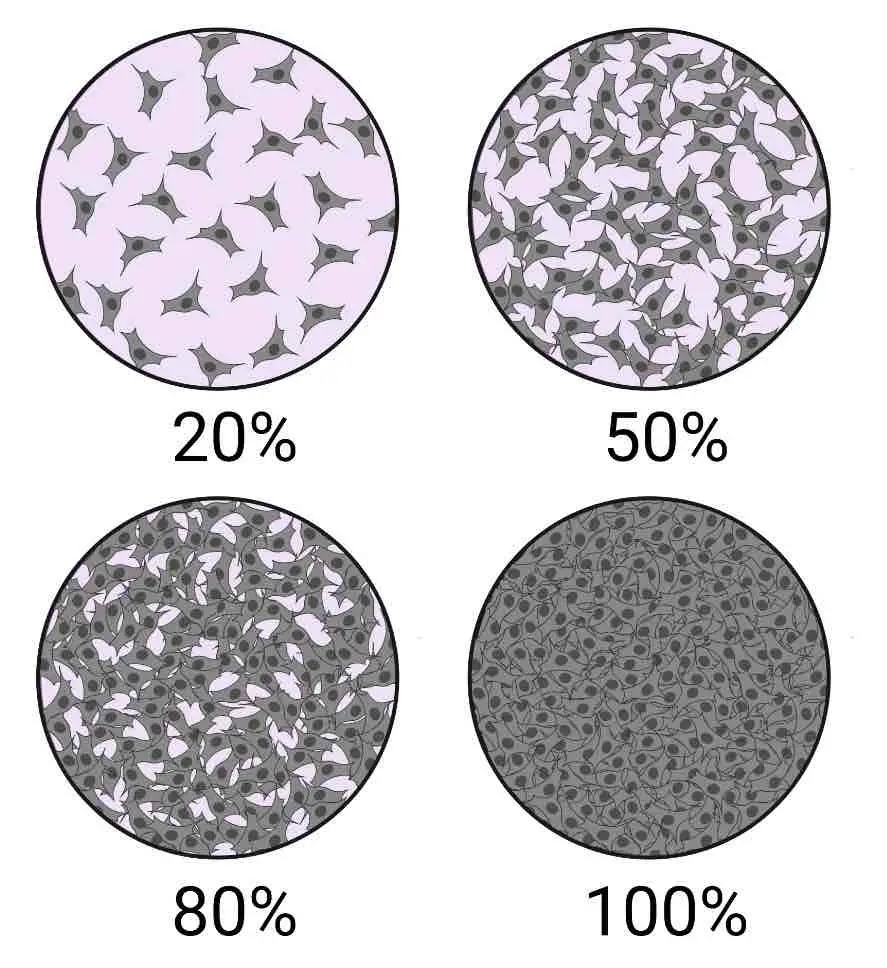
[In this image] Cellular Confluence.
Cellular confluence generally refers to the percentage of the culture vessel inhabited by attached cells. For example, 100% cellular confluence means the surface area is completely covered by cells, whereas 50% confluence means roughly half of the surface is covered.
Image source: Technology Networks Cell Science
To subculture adherent cells, the first step is to detach them from the surface of the culture container. This can be done using enzymes or mechanical methods such as shaking or scraping. Primary cells typically require the use of proteolytic enzymes, like trypsin/EDTA, to break the protein bonds that anchor them to the culture dishes. Once the cells are detached, they are collected as a single-cell suspension and separated by centrifugation. The resulting cells can then be re-seeded into new cell culture dishes with fresh growth medium.
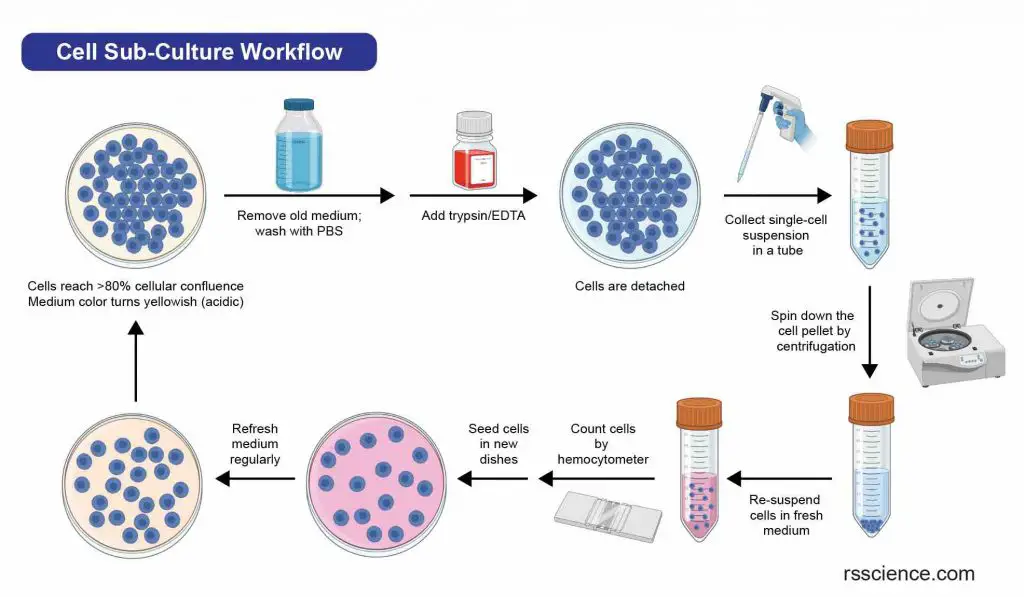
[In this image] Flowchart of a typical cell passaging procedure.
As a measure of cell health, researchers may count the number of cells between two passages. This can be done using a hemocytometer and viability staining with trypan blue. Check “How to Use a Hemocytometer to Count Cells” for more information.
Cryopreservation
If there is an excess of cells during subculturing, they can be preserved by storing them at temperatures below -130°C through a process called cryopreservation. To prevent ice crystals from forming and damaging the cells, they are typically mixed with a protective agent such as dimethyl sulfoxide (DMSO) or glycerol. These cryopreserved cells can be stored until they are needed for future use.
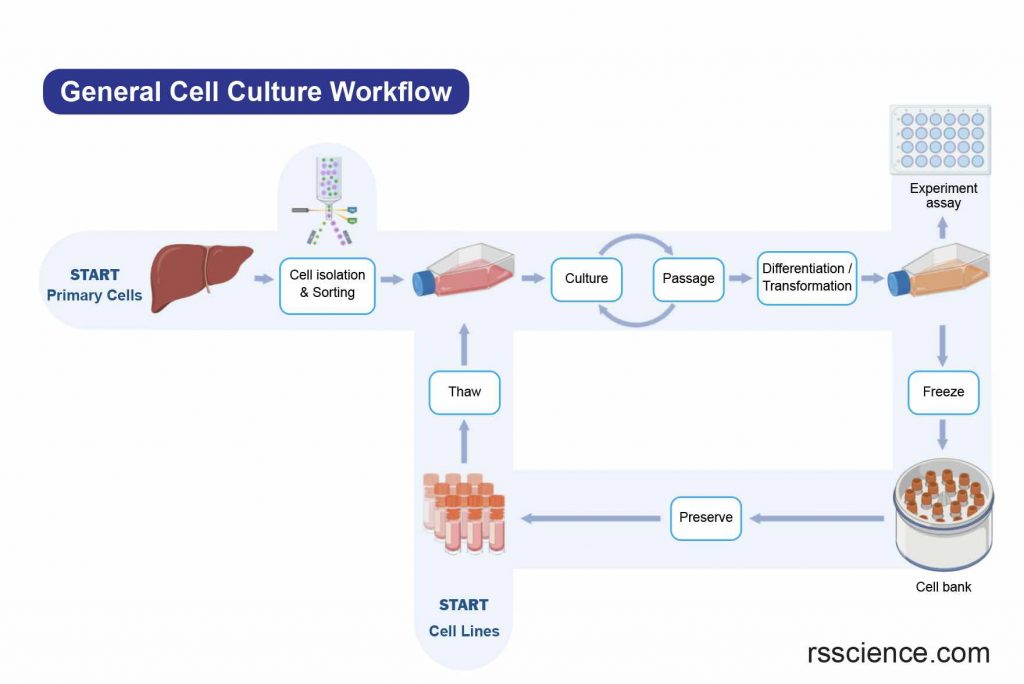
[In this image] A flowchart of a generalized cell culture process.
Table. Basic equipment required for cell culture.
Cell culture hood
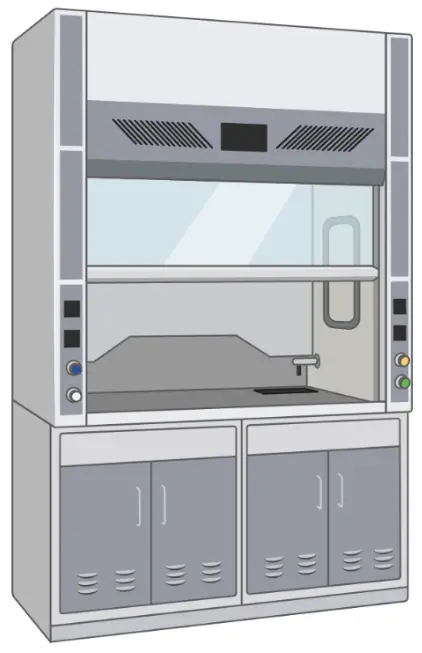
Also known as the Laminar flow hood, which provides an ultra-clean working place for aseptic handling of cell culture.
Incubator
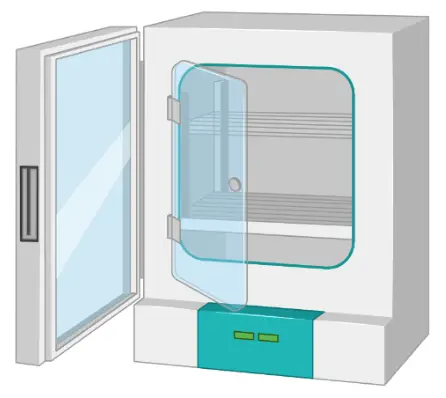
Cell incubators enable optimal growth conditions by controlling the temperature, humidity, and CO2 level.
Inverted microscope
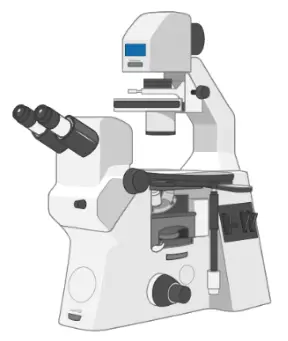
An inverted microscope (4X, 10X, 20X, and 40X objective lenses) is essential to monitor the healthiness and confluence of cells.
Centrifugation
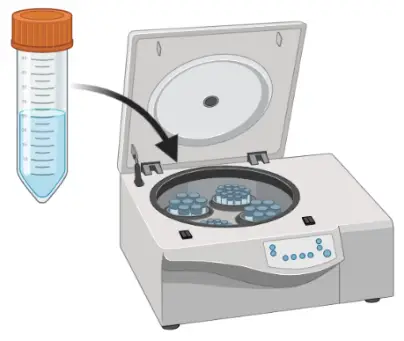
A centrifuge can generate a centrifugal force (usually ~ 200 x g) and is needed to spin down suspended cells.
Water Bath
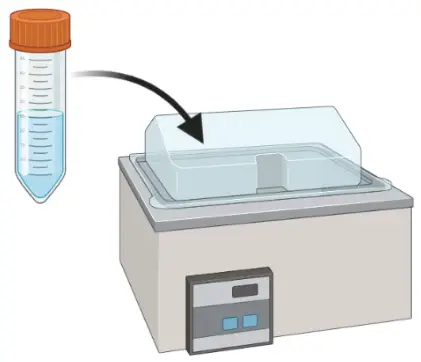
A water bath set to 37oC is commonly used for the pre-warming medium before use and for thawing frozen cells.
Fridge and Freezer
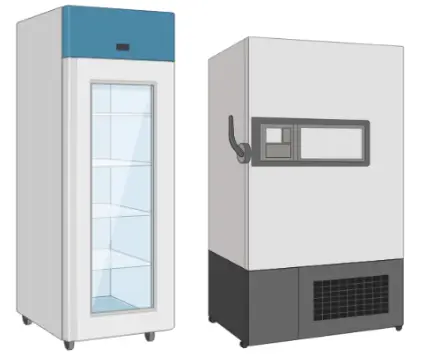
For the storage of cell culture media (2 – 8oC) and other cell culture reagents (-20oC).
Liquid nitrogen tank
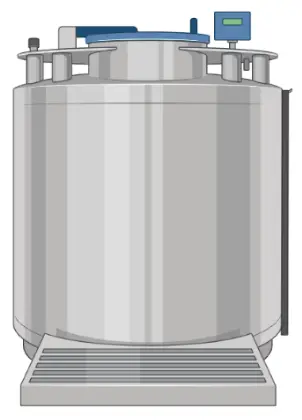
For the long-term cryopreservation of cells at temperatures below -130oC.
Hemocytometer
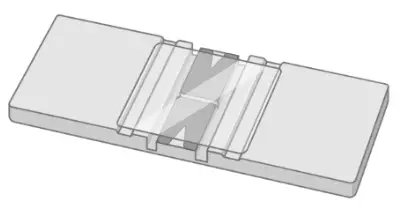
A hemocytometer is a counting-chamber device used to count cell numbers.
Pipet Aid
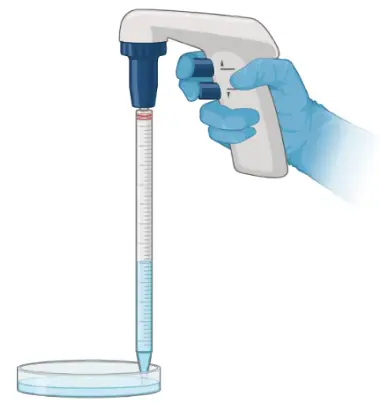
With a pipet aid and pipets (i.e., 2, 5, 10, 25 mL), researchers can transfer fluid precisely for medium changing or cell passaging.
Pipettes
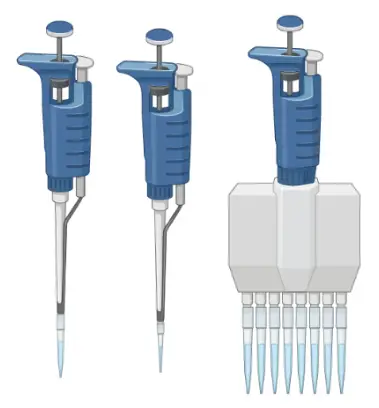
Pipettes are used to dispense small volumes of liquid (usually, ranging from 1µL to 1mL) using single-use plastic pipette tips.
Cell Culture Vessels
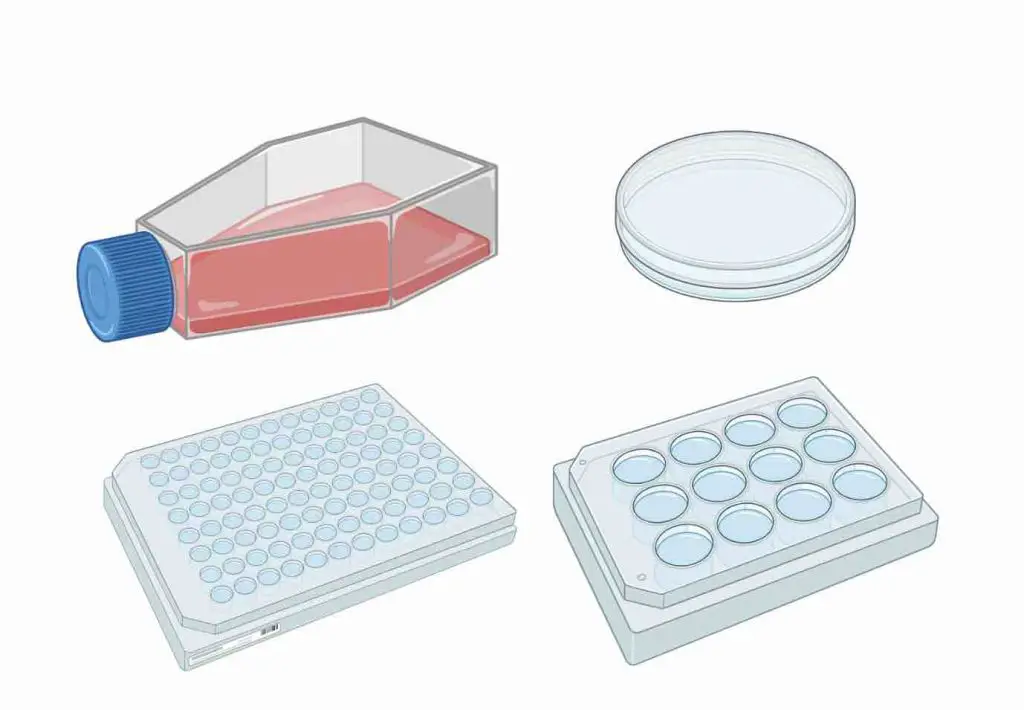
Culture-grade plasticware treated for adherent cell attachment. There are options in a variety of sizes and shapes.
Consumables
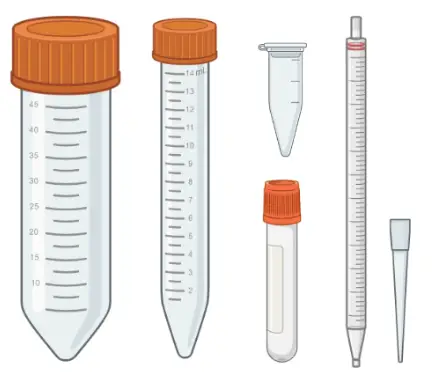
Common sterile, single-use consumables for cell culture include various tubes, pipets, and pipette tips.
Personal protective equipment
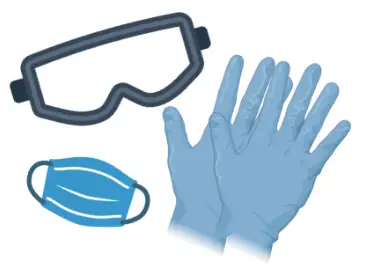
Personal protective equipment (i.e., lab coat, gloves, eyewear, mask) is required according to the biosafety levels.
Images were created with BioRender.com.
Source of Cells – Primary Cell v.s. Cell Lines
There are various ways to classify cell culture. One way to classify cell culture is by the source of the cells. The cells may be removed from the tissue directly and disaggregated by enzymatic or mechanical means before cultivation. Or they may be derived from a cell line or cell strain that has already been established.
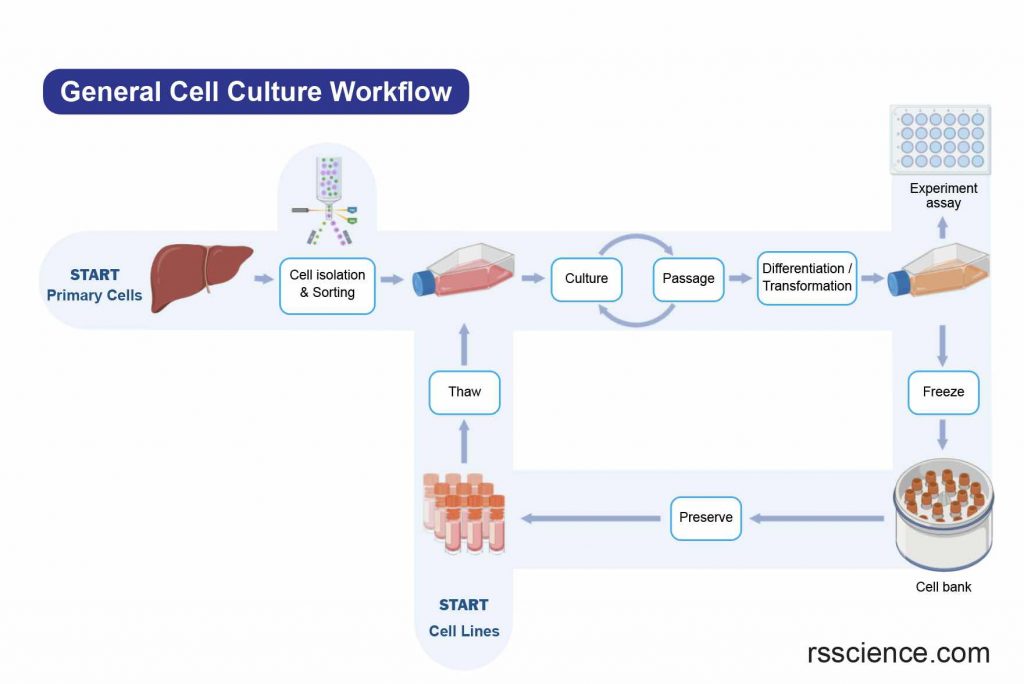
[In this image] The general workflow of cell culture.
The cell sources can be from freshly isolated cells (Primary cells) or from established cell banks (Cell lines).
Primary cells
Primary cells are those that are directly extracted from a tissue or organ and are usually used in a single experiment. They are often preferred in research studies that require the use of cells that closely resemble those found in the body. Primary cells are more representative of the original tissue, leading to more accurate results. However, their lifespan is limited, as they can only divide a few times (one to two times) in cell culture.
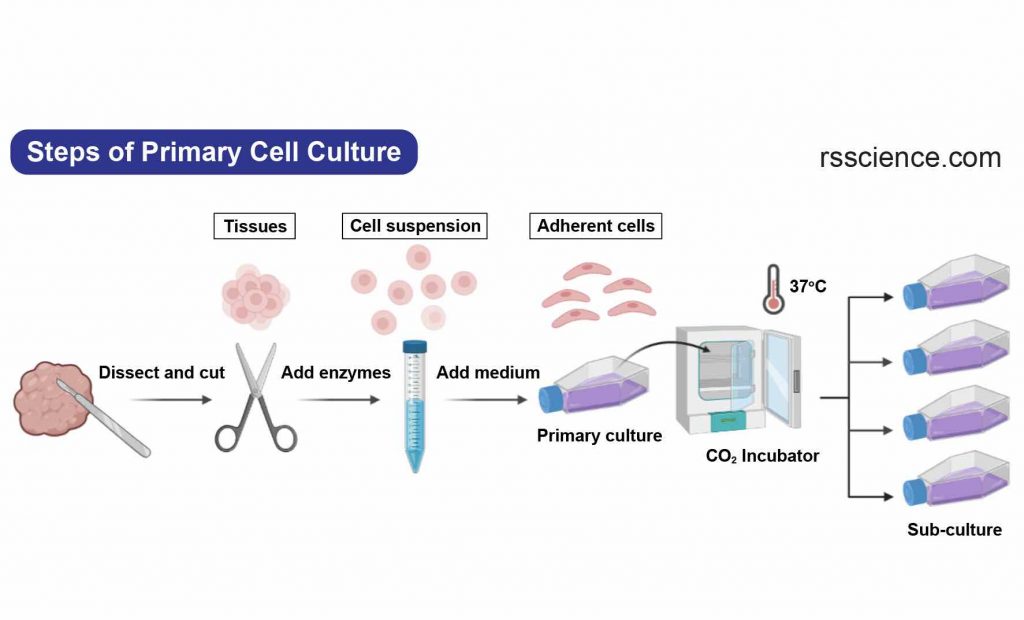
[In this image] The steps to establish a primary cell culture.
Cell lines
Cell lines, on the other hand, are cells cultured in the laboratory for an extended period of time and have the ability to grow and divide indefinitely. Cell lines are derived from primary cells and are often used in research and other applications because they are easy to grow and maintain in the laboratory.
Normal cells have a limited capacity for proliferation and will eventually stop dividing, a process known as senescence. However, some cell lines can become immortal through a process called transformation. Transformation can occur naturally, as is the case with cancer cells, or it can be induced by external factors such as chemicals or viruses.
When a finite cell line undergoes transformation and gains the ability to divide indefinitely, it becomes a continuous cell line. Continuous cell lines are able to divide and proliferate indefinitely, unlike normal cells which have a limited capacity for proliferation.
Cell Line | Picture | Cell Type | Species | Tissue |
HEK 293 | ![]() | fibroblast | human | embryonic kidney |
BALB/3T3 | ![]() | fibroblast | mouse | embryo |
C2C12 | ![]() | myoblast | mouse | muscle |
CHO-K1 | ![]() | epithelial | hamster | ovary |
HeLa | ![]() | epithelial | human | cervix carcinoma |
HEp-2 | ![]() | epithelial | human | larynx carcinoma |
HepG2 | ![]() | liver | human | hepatocarcinoma |
HL-60 | ![]() | lymphoblast | human | promyelocytic leukemia |
HUVEC | ![]() | endothelial | human | umbilical cord |
MCF7 | ![]() | epithelial | human | breast adenocarcinoma |
PC12 | ![]() | neuronal | rat | adrenal gland |
Pluripotent stem cells
Pluripotent stem cells have characteristics of both cell lines and primary cells. They are called “pluripotent” because they have the potential to give rise to any cell type. In an undifferentiated status, pluripotent stem cells can divide and proliferate indefinitely. This makes them similar to cell lines.
However, unlike cell lines, which become immortal through transformation, pluripotent stem cells have the ability to divide indefinitely due to their nature as stem cells. This means that they also retain the properties of normal primary cells.
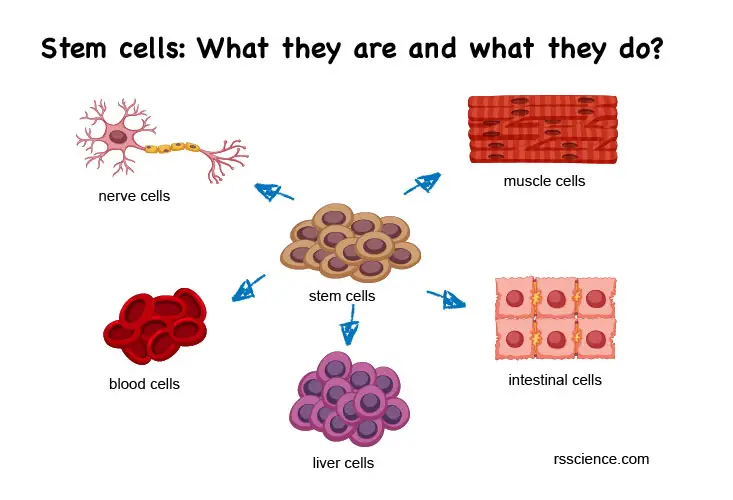
[In this image] Pluripotent stem cells are a type of stem cell that has the ability to differentiate into any type of cell in the body.
There are two main types of pluripotent stem cells: embryonic stem cells (ESCs), which are derived from the inner cell mass of a blastocyst during early embryonic development, and induced pluripotent stem cells (iPSCs), which are adult cells that have been genetically reprogrammed to behave like pluripotent stem cells.
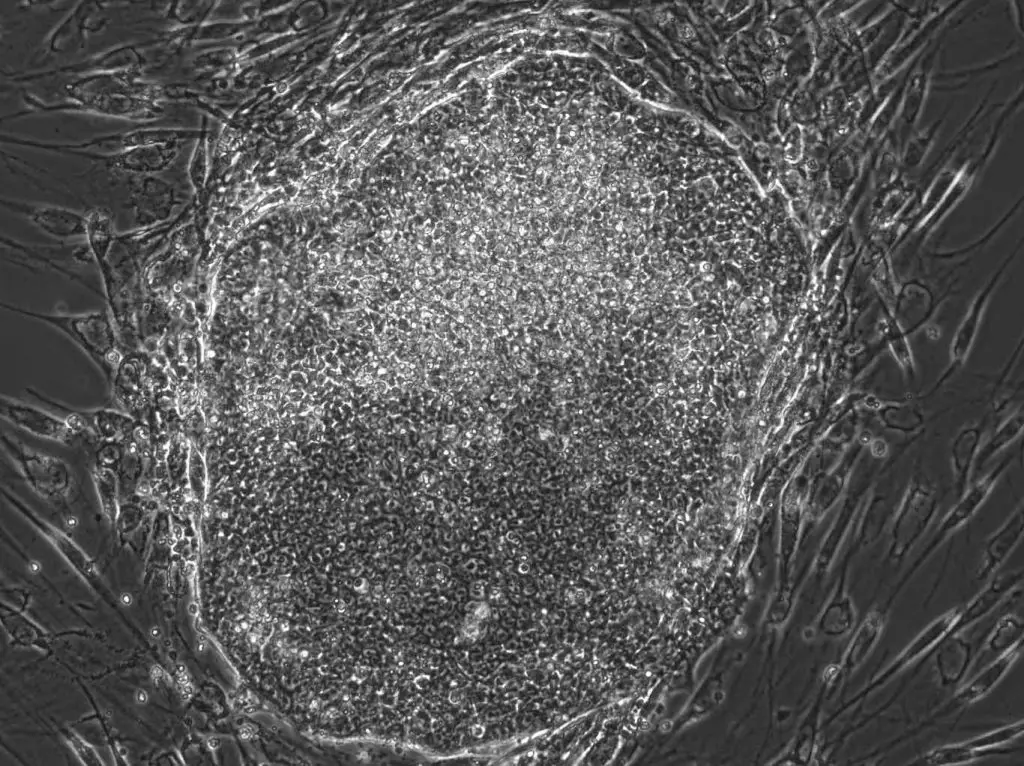
[In this image] A human iPSC colony I made in a laboratory. The original cells are human fibroblast cells from the skin. They are reprogrammed to iPSCs by “Yamanaka factors” in 3 weeks.
Extended Reading: What is an induced pluripotent stem cell (iPSC)?
Table. Comparison between primary cells, cell lines, and pluripotent stem cells:
Cell types | Primary cells | Cell lines | Pluripotent stem cells |
Origin | directly isolated from a tissue or organ | derived from primary cells through transformation | derived from embryonic tissues or induced from primary cells |
Lifespan | limited lifespan and will eventually stop dividing and die | can grow and divide indefinitely | can grow and divide indefinitely if not differentiate |
Biological Relevance | high | low | high |
Consistency | medium | high | high |
Cell culture conditions | widely depended on the cell types | usually adapted to the usage of standard culture media | use specialized stem cell culture media |
Ease-of-Use | needs optimized culture conditions | well-established conditions and protocols | well-established conditions and protocols; but more vulnerable than cell lines |
Time & Expense | slower cell growth and higher cost | faster cell growth and lower cost | faster cell growth and higher cost |
Genetic stability | exhibit genetic changes over time due to the effects of cell culture | more genetically stable | more genetically stable |
Representative of the original tissue | more representative of the original tissue from which they were derived | have undergone genetic and phenotypic changes due to their prolonged growth in culture | representative of the pluripotent status of stem cells |
Applications | for studies that require the use of cells that are representative of the original tissue | commonly used in research and other applications where ease of maintenance and long-term growth are important | for studies of cell differentiation; cell sources for cell therapy and regenerative medicine |
Morphology of Cells in Culture – Observing cultured cells under a microscope
Cells in culture can be divided into three basic categories based on their shape and appearance (i.e., morphology).
Fibroblastic (or fibroblast-like) cells
Fibroblastic (or fibroblast-like) cells are elongated spindle-shaped, and attached to a substrate to grow.
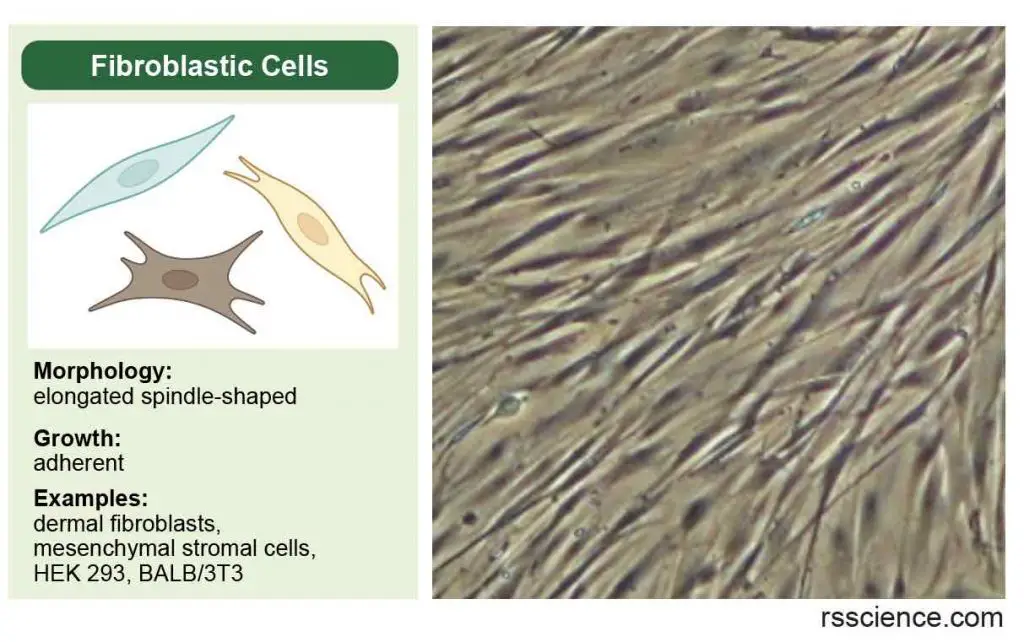
[In this image] Fibroblastic (or fibroblast-like) cells have an irregular, elongated spindle shape. They form our connective tissues.
Epithelial-like cells
Epithelial-like cells are polygonal in shape with more regular dimensions and grow attached to a substrate in discrete patches.
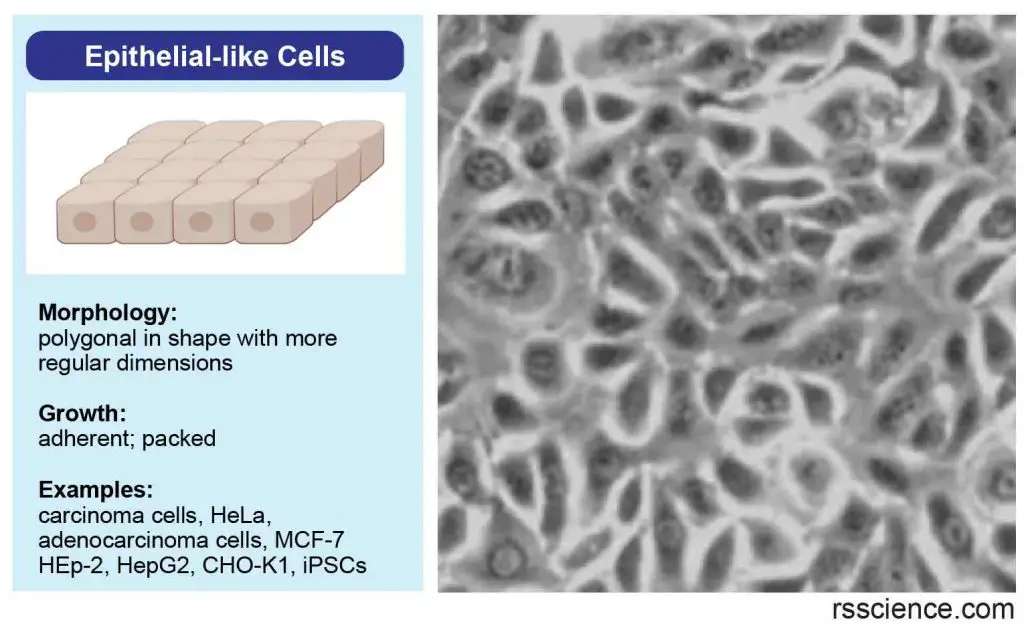
[In this image] Epithelial-like cells have a more regular, polygonal shape. They form our epithelial tissues.
Lymphoblast-like cells
Lymphoblast-like cells are spherical in shape and usually grown in suspension without attaching to a surface.
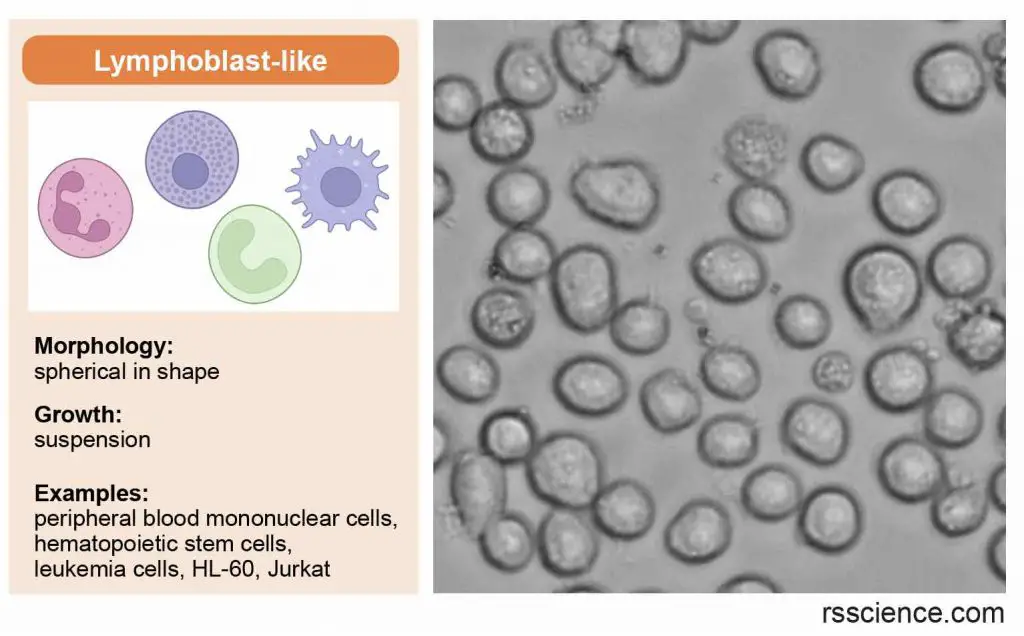
[In this image] Lymphoblast-like cells are spherical in shape and usually grown in suspension. They come from our blood.
Applications of Cell Culture
Cell culture is a widely used technique in biological and medical research, as it allows researchers to study cells in a controlled environment. Cell culture has a variety of applications, including the production of vaccines, the study of cell growth and development, the study of the effects of drugs and other chemicals on cells, and the production of genetically modified cells for use in research or therapy. In addition, cell culture is used to produce cells and tissues for use in transplantation and regenerative medicine. It is also used in the production of biopharmaceuticals, such as monoclonal antibodies and other protein-based drugs.
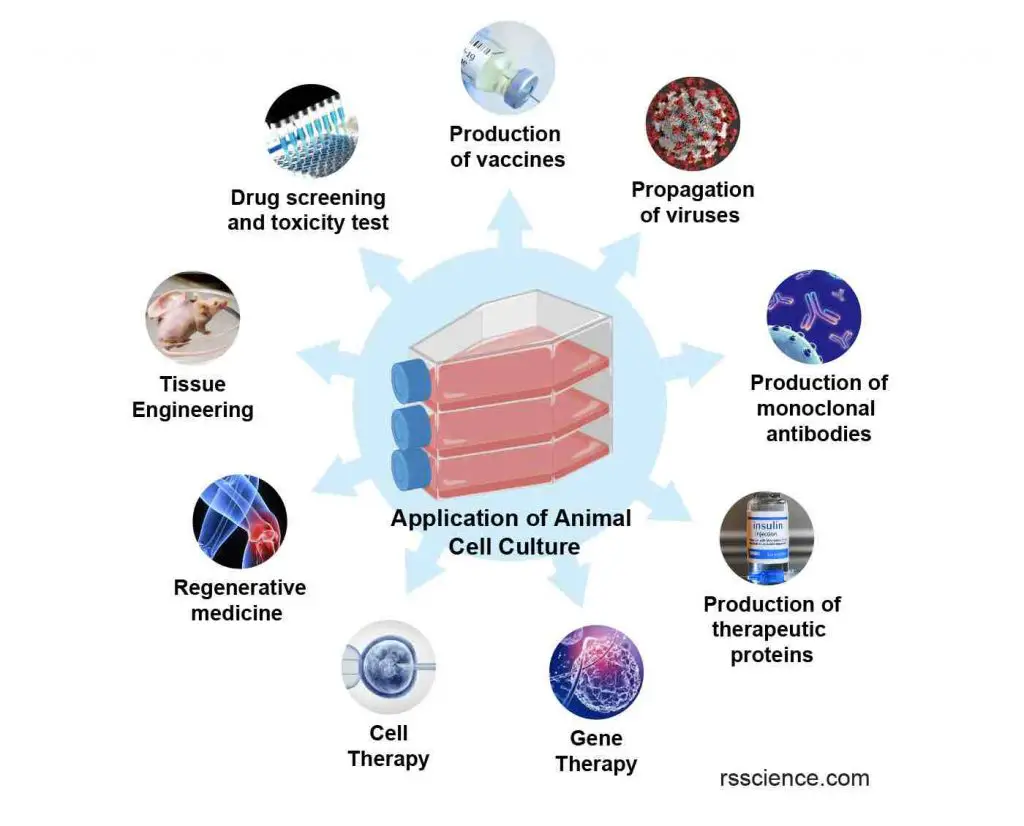
[In this image] Various applications of cell culture technique in basic and medical research, drug development, vaccine and therapeutic protein production, gene & cell therapy, and regenerative medicine.
Cell and Molecular biology
Cell culture allows scientists to study many cellular processes, including programmed cell death (apoptosis), cell proliferation, cell activation, cell cycle, gene expression and cell signaling.
Extended Reading: Fluorescence Microscope as a powerful tool to study cell biology
Medical sciences
Cells from patients’ tissues allow us to study the causes and treatment of diseases.
Cancer research
Primary cells can be exposed to radiation, chemicals, and viruses to make them cancerous. Thus, the cause of cancer and the altered signaling pathways can be studied. Cell culture can also be used for the determination of effective drugs for cancer cells.
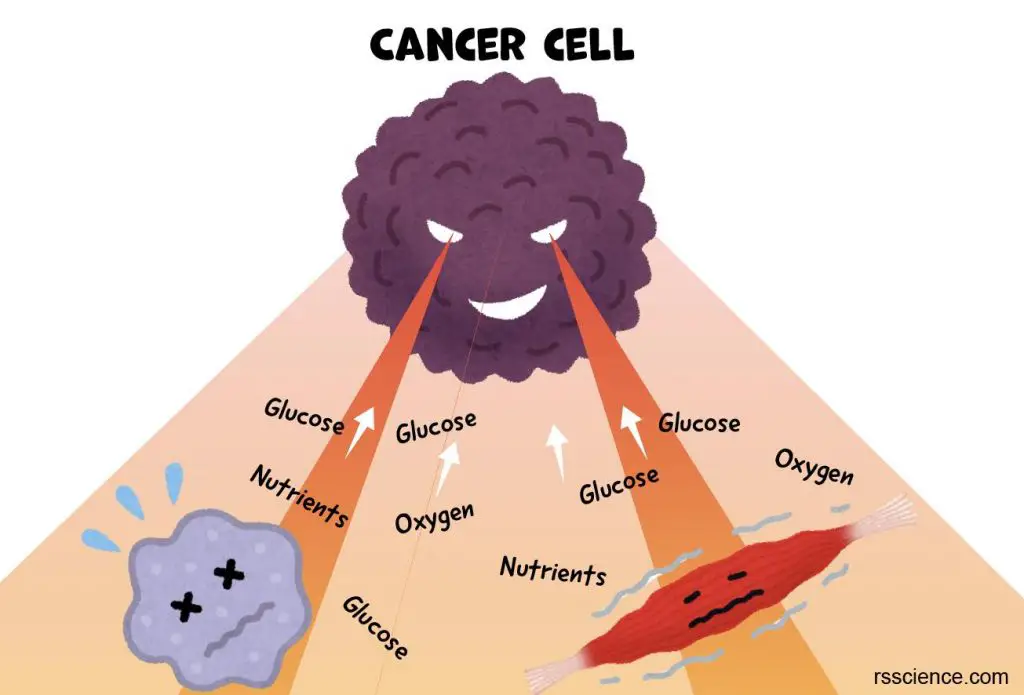
[In this image] Cancer cells plunder the nutrients from surrounding cells and tissues to sustain the rapid growth of tumors.
Drug screening and toxicity test
Cell culture provides a quick and easy testing platform for us to know the effects of an unknown chemical. Scientists can tell if this compound has the potential to become a drug or if it is too toxic for human bodies. Using cell culture to test drugs can significantly reduce the usage of animal experiments.
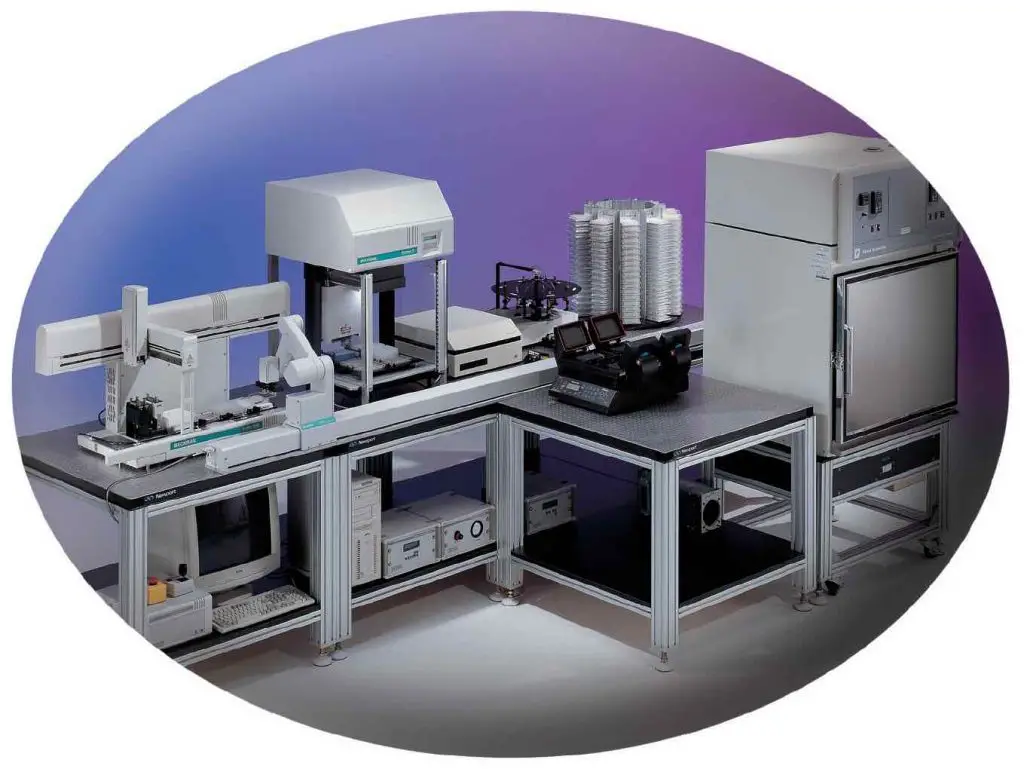
[In this image] A high throughput screening (HTS) system with integrated incubators, plate stackers, 96 well pipettes, serial diluters, imagers, and robotic arms. Big pharmaceutical companies routinely use these systems to screen drug candidates.
Image source: Drug discovery world
Vaccines
Cell culture can service as the hosts of viruses. Scientists can propagate viruses and use them to produce vaccines.
Biotechnology
Cell lines can be engineered to produce therapeutic agents, including enzymes, monoclonal antibodies, growth factors, biopesticides, etc. The most known industrial cell line is the Chinese hamster ovary (CHO) cell.
Regenerative medicine
Cell culture technique can be used to propagate cells for cell transplantation and tissue engineering.
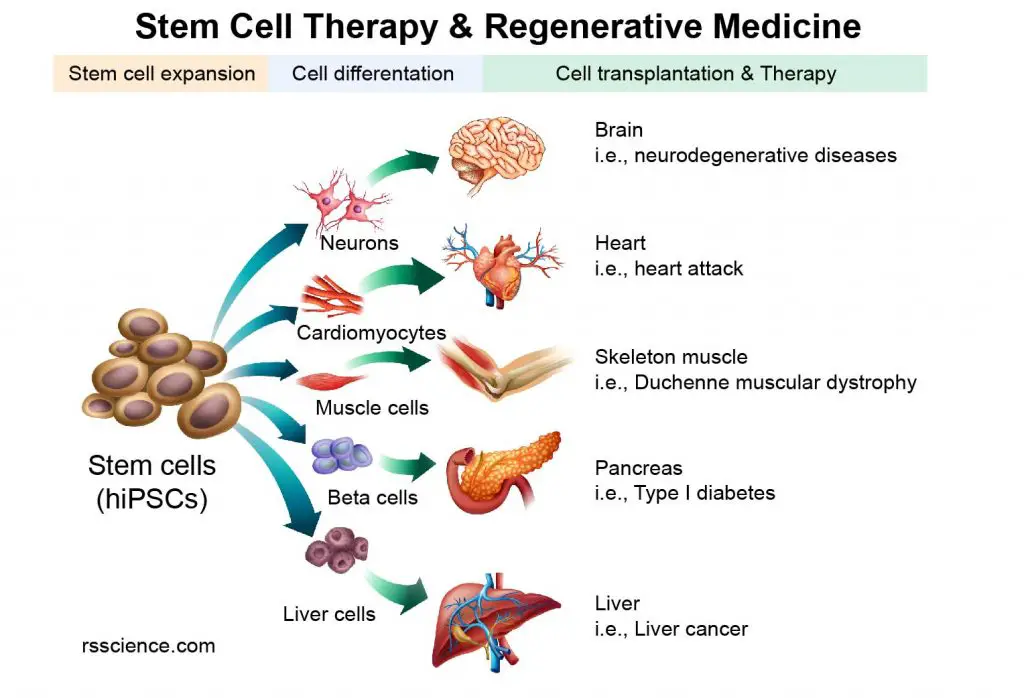
[In this image] The concept of Stem Cell Therapy and Regenerative Medicine.
Gene therapy
The cells of patients with certain genetics diseases can be “fixed” in cell culture (through a technology called gene editing) and transplanted back to replace the diseased cells.
Cancer therapy
Patients’ immune cells (mainly T cells and NK cells) can be “educated” to recognize cancer antigens in cell culture (by CAR-T technology). These “trained” immune cells can be transfused back to patients to kill the cancer cells.
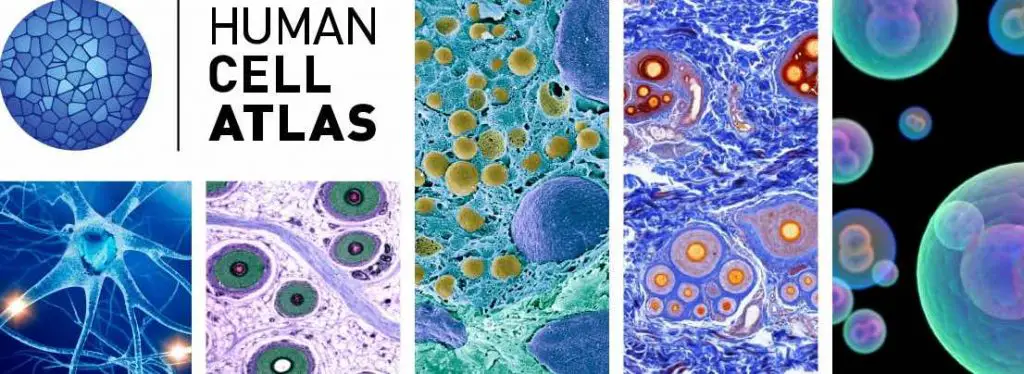
[In this image] To know more about our cells, Broad Institute (Cambridge, MA) initiated the Human Cell Atlas Project aiming to bring together an international community of scientists and to create a comprehensive reference map of all human cells.
Image source: Broad Institute
Culture of non-mammalian cells
In addition to the culture of mammalian cells for biomedical research, cells from other living organisms can be cultured for many applications.
Plant cell and tissue culture
Plant tissue culture involves growing plants in a laboratory setting using small pieces of plant tissue. This technique allows for the rapid and efficient production of large numbers of genetically identical plants. It is often used for plants that are difficult to propagate through other methods, such as seeds or cuttings. In addition to being a useful tool for plant propagation, plant tissue culture is also important in the creation of genetically modified plants.
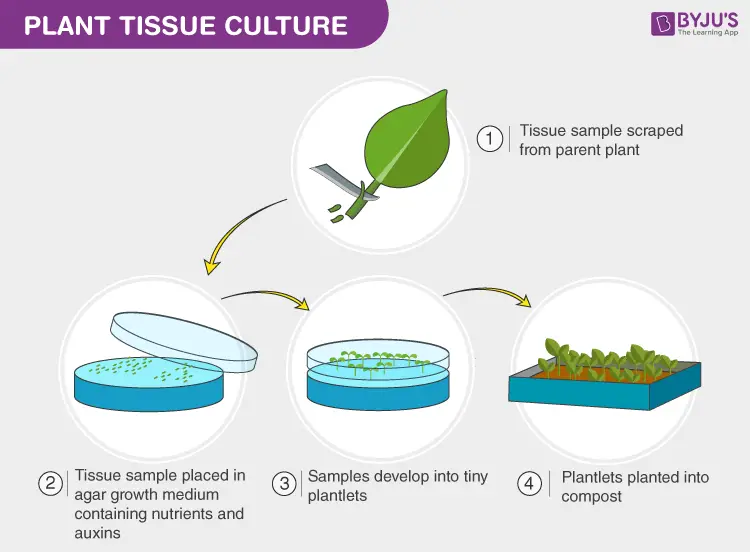
[In this image] Using plant tissue culture can rapidly propagate produce large numbers of plants without their seeds.
Image source: BYJU’S
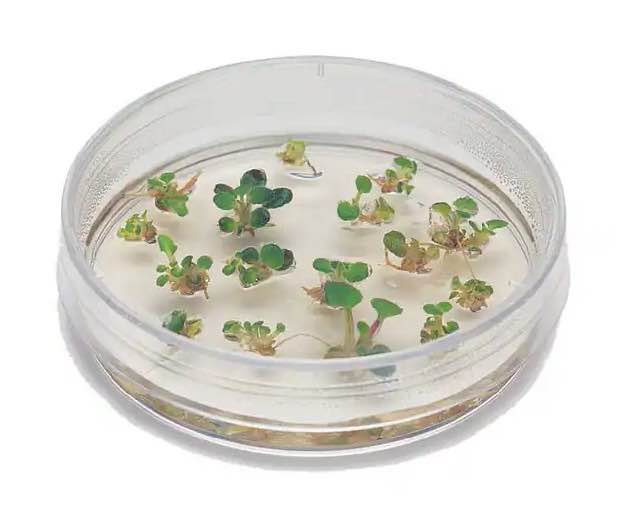
[In this image] Many small new plants grow in a dish with nutrient-rich agar medium.
Image source: Fisher Scientific
Insect cell culture
Insect cells can be cultured in a nutrient-rich media, which allows them to grow and divide. Insect cells are relatively easy and inexpensive to culture compared to mammalian cells, making them popular for producing proteins, vaccines, and other products for research or industrial purposes.
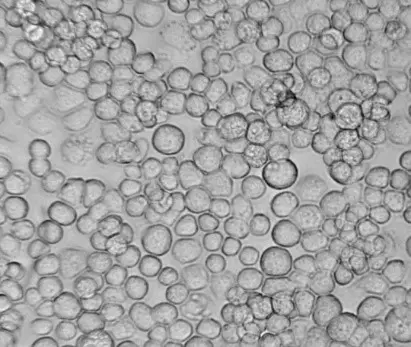
[In this image] Sf9, an insert cell line derived from the armyworm Spodoptera frugiperda, is commonly used for industrial recombinant protein production.
Image source: Oxford expression technologies
Bacterial and yeast culture
Bacteria and yeasts are typically grown in small quantities on a solid medium containing nutrients, such as agar. For large-scale cultures, these cells are grown suspended in a nutrient broth. This method allows for the proliferation and study of bacteria and yeasts in a laboratory setting.
Extended Reading: How to See Bacteria on Your Hand (Bacteria Handprint)
Viral culture
Viruses cannot live alone. In order to culture viruses, host cells of mammalian, plant, fungal, or bacterial origin are required. These host cells provide an environment where the virus can grow and replicate. Large-scale cultivation of viruses is used to produce vaccines.
History of Cell Culture
The concept of cell culture can be traced back to the early 1800s when scientists first began to study cells and tissues under the microscope. However, it wasn’t until the late 1800s and early 1900s that the first successful cell cultures were established.
One of the earliest successful attempts at cell culture was made in 1885 by Wilhelm Roux, a German scientist. He was able to grow cells from the spinal cord of a chicken embryo in a warm saline solution for several days, which established the basic principle of tissue culture.
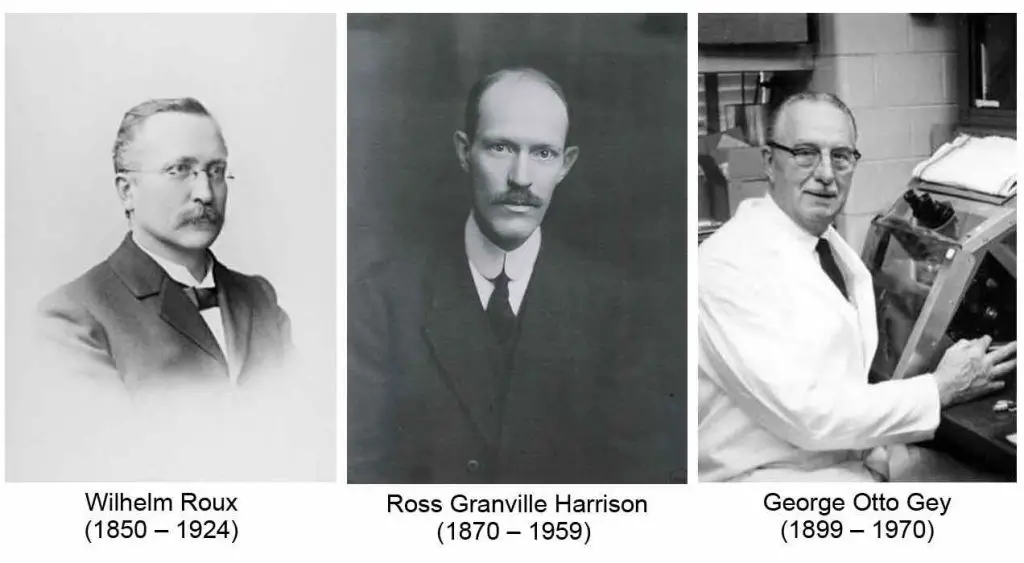
[In this image] Wilhelm Roux, Ross Granville Harrison, and George Otto Gey
Image source: wiki
In the early 20th century, Ross Granville Harrison, an American biologist, made significant progress in understanding the requirements for growing cells in culture, including the importance of using a sterile environment and a nutrient-rich medium. This marked the beginning of modern cell culture techniques.
In the 1930s, George Gey and Margaret Gey developed a method for growing human cells in culture using a serum-based medium, which allowed them to grow cells from a variety of tissues and organs. This method, known as the Gey method, became widely used in cell culture research and paved the way for the development of many important medical treatments, including vaccines and cancer therapies.
In 1951, Gey’s laboratory isolated cells from a cervical tumor removed from Henrietta Lacks. These cells, known as HeLa cells, were found to be particularly hardy, and once Gey realized this, he began sharing them with scientists all over the world. The widespread use of the HeLa cell line played a significant role in the advancement of cell culture techniques.
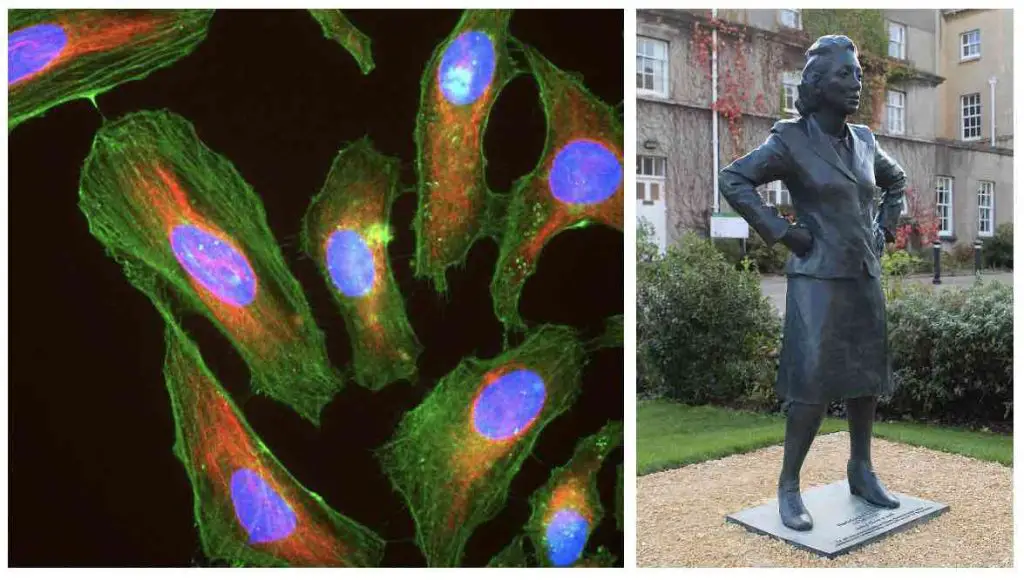
[In this image] Left: Immunofluorescence image of HeLa cells grown in tissue culture and stained with antibody against actin in green, vimentin in red, and DNA in blue. Right: Statue of Henrietta Lacks.
Image source: wiki
Cell culture techniques were also advanced significantly in the 1940s and 1950s to support research in virology. Because viruses require cells as hosts, growing viruses in cell cultures allowed for the preparation of purified viruses for use in the production of vaccines. In 1954, John Franklin Enders, Thomas Huckle Weller, and Frederick Chapman Robbins were awarded the Nobel Prize for their discovery of a method of growing viruses in cell cultures. Their work paved the way for further research in the field and the development of numerous vaccines.
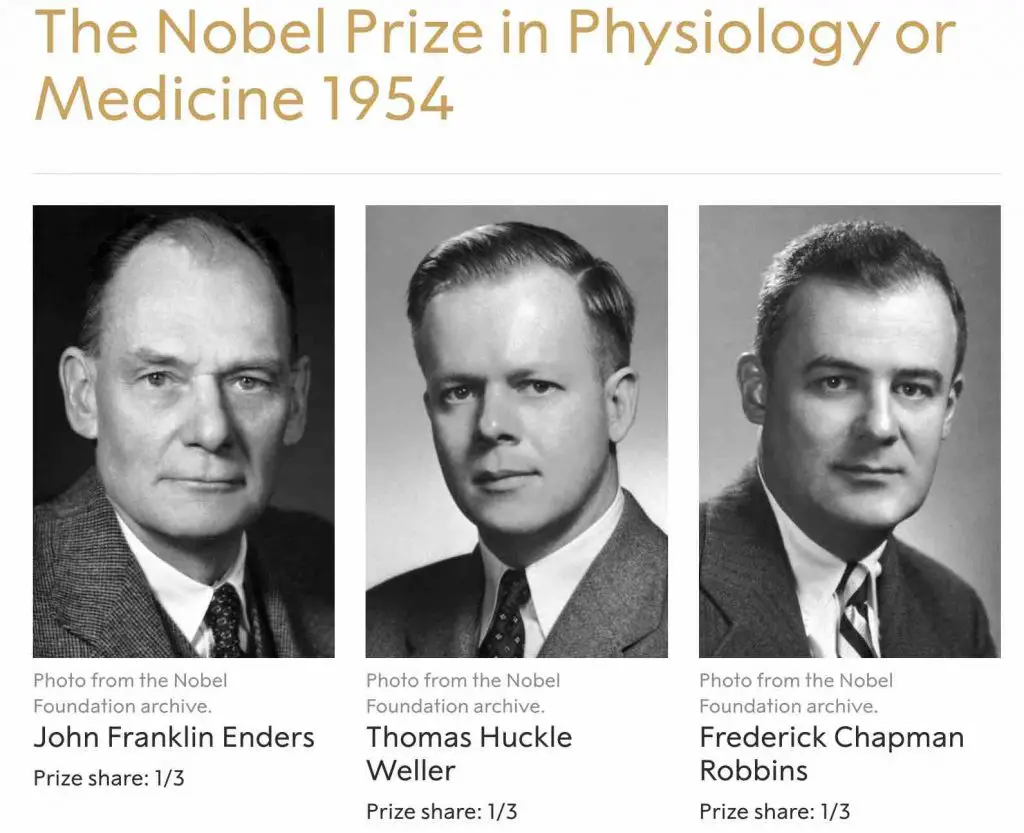
[In this image] The Nobel Prize in Physiology or Medicine 1954 was awarded jointly to John Franklin Enders, Thomas Huckle Weller, and Frederick Chapman Robbins for their discovery of the ability of poliomyelitis viruses to grow in cultures of various types of tissue.
Image source: The Nobel Prize
Cell culture techniques have undergone significant refinements and improvements over the years, and today, they are widely used in various fields of science, including cell biology, genetics, and molecular biology. Cell culture is also used in the production of drugs, vaccines, and other medical treatments, as well as in the development of cell-based therapies and tissue engineering. The continued advancement of cell culture techniques has greatly impacted and benefited various scientific and medical fields.
Summary
1. Cell culture is a technique used in the laboratory to grow and maintain cells in a Petri dish or flask.
2. Cell culture allows scientists to study the growth, development, the function of specific genes, and other characteristics of cells under controlled conditions.
3. Cell culture is a valuable tool in many areas of research, including molecular biology, biochemistry, genetics, and pharmacology.
4. Cell culture requires a nutrient-rich medium, a flat surface for cells to anchor (excluding blood cells), a neutral pH of 6.8 to 7.4, a body temperature of 37°C (or 98.6°F), isotonic osmotic pressure, a source of oxygen, and regular removal of waste.
5. The cell sources can be from freshly isolated cells (Primary cells) or from established cell banks (Cell lines).
6. Cells in culture can be divided into three basic categories based on their shape and appearance (i.e., morphology): fibroblast-like cells, epithelial-like cells, and lymphoblast-like cells.
7. In addition to the culture of mammalian cells for biomedical research, cells from plants and insects can be cultured for creating genetically modified plants and protein production, respectively.
8. In 1885, a German scientist, Wilhelm Roux, was able to grow cells from the spinal cord of a chicken embryo in a warm saline solution for several days, which established the basic principle of tissue culture.
9. Viruses require cells as hosts. Cell cultures allow the preparation of purified viruses for use in the production of vaccines. The Nobel Prize in Physiology 1954 was awarded jointly to John Franklin Enders, Thomas Huckle Weller, and Frederick Chapman Robbins for their discovery of the ability of poliomyelitis viruses to grow in cultures of tissue.