Cytoplasmic streaming, also called Protoplasmic Streaming or Cyclosis, plays an important role in cell processes bacause it promotes the movement of the fluid substance (cytoplasm) within a cell.
[In this video] Cytoplasmic streaming in Elodea cells under the microscope.
This article covers
Can I see cytoplasmic streaming under my microscope?
Of course, cytoplasmic streaming is easy and fun to look at under a compound microscope. The key is to look at the right specimens. Elodea (or waterweed) is an aquatic plant and the best specimen to study cytoplasmic streaming for beginners.
[In this video] Looking at chloroplasts moving by cytoplasmic streaming in plant cells (Elodea) – DIC microscope/ 1250x.
Materials
- Live Elodea plants – can be easily found in small ponds or aquarium shops.
- Compound microscope
- Scissors and tweezers
- Microscope slide and coverslip
Instructions
- Cut a small leaf of Elodea.
- Prepare a wet mount slide.
- Start from a lower magnification (5-10x) to find the focus of cells.
- Switch carefully to higher magnification (20-40x) to identify the chloroplasts.
- Keep the slide under the light illumination for a few minutes. This can warm up the cells and activate the cytoplasmic streaming.
- Now you should be able to see the chloroplasts cycling around the central vacuoles of cells.
Things to look
- If you have a video camera mounted on the microscope, could you measure the speed of cytoplasmic streaming?
- Does cytoplasmic streaming occur in the same direction in all cells or is the movement random?
- Does the speed of cytoplasmic streaming change? Try different light intensity or add chemicals (eg, sugar, salt, vinegar …)
- Does cytoplasmic streaming also happen in other cells? Try other plant cells (like onion skins, leaf peels …), microorganisms (like paramecium, amoeba, green alga …).
Why is cytoplasmic streaming important?
Cytoplasmic streaming is important in plant cells and large single-celled animal cells because passive diffusion is not adequate for nutrient molecule distribution in large cells. Cytoplasmic streaming can efficiently transport essential biological molecules across a big cell.
In plant cell, cytoplasmic streaming can be used to distribute chloroplasts for maximum light absorption for photosynthesis. Cytoplasmic streaming also allows the long-distance transportation of biological molecules across different cells.
What are the biological functions of cytoplasmic streaming?
1. Enhanced nutrient transport in large cells
Cytoplasmic streaming is important for single-celled protists because they are relatively large cells (compared to individual cells in multicellular organisms). For these large cells (bigger than 100 µm), passive diffusion may not be enough to transport molecules efficiently throughout the entire cell. The motion of cytoplasmic streaming creates a current inside the cell and promotes the transportation of nutrients, proteins, and organelles for extensive distances. It helps the exchange of materials inside these big cells significantly.
Paramecium
For example, Paramecium is a pretty large cell (250-300 µm) and relies on cytoplasmic streaming to exchange nutrients and metabolites between the cytoplasm and organelles. Cytoplasmic streaming circulates the cytoplasm and organelles around the paramecium cell. The food vacuoles move around via cytoplasmic streaming to distribute the nutrients in the cell.
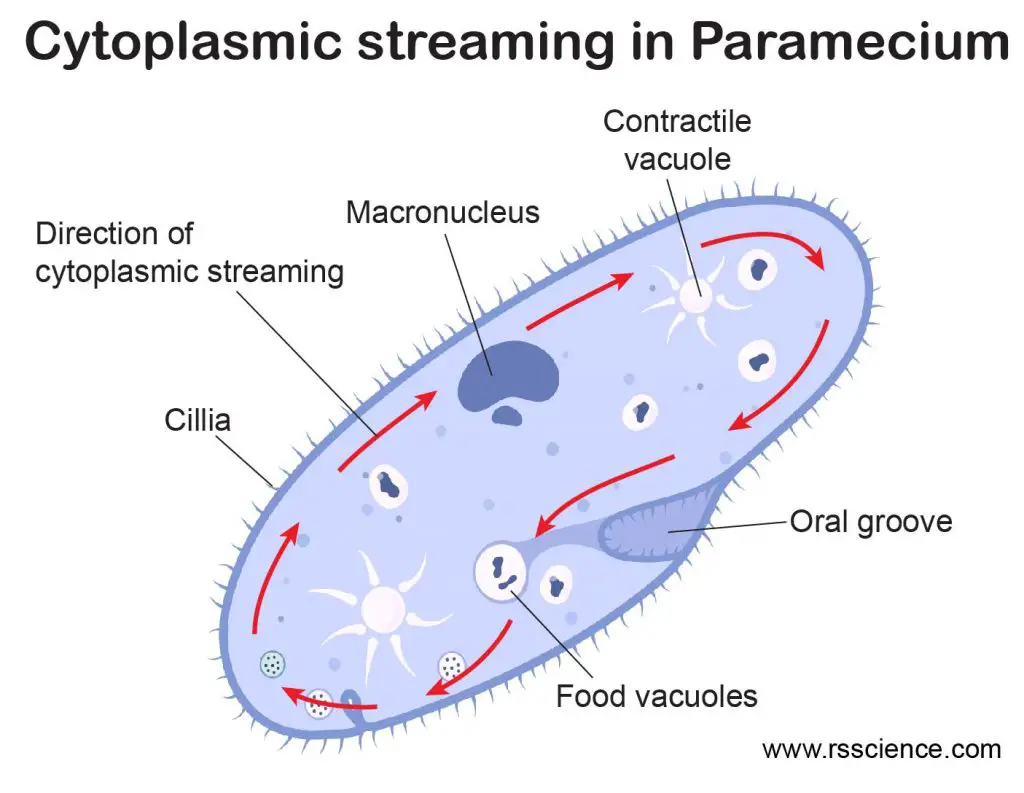
[In this figure] Cytoplasmic streaming in Paramecium.
Cytoplasmic streaming circulates the cytoplasm and organelles such as food vacuoles around the cell.
Amoeba
Another example is the amoeba. Amoeba move by extending their pseudopods. This process involves a significant change in amoeba cell shape. Cytoplasmic streaming can efficiently bring the content of cells to fill up the new space after the movement of pseudopods.
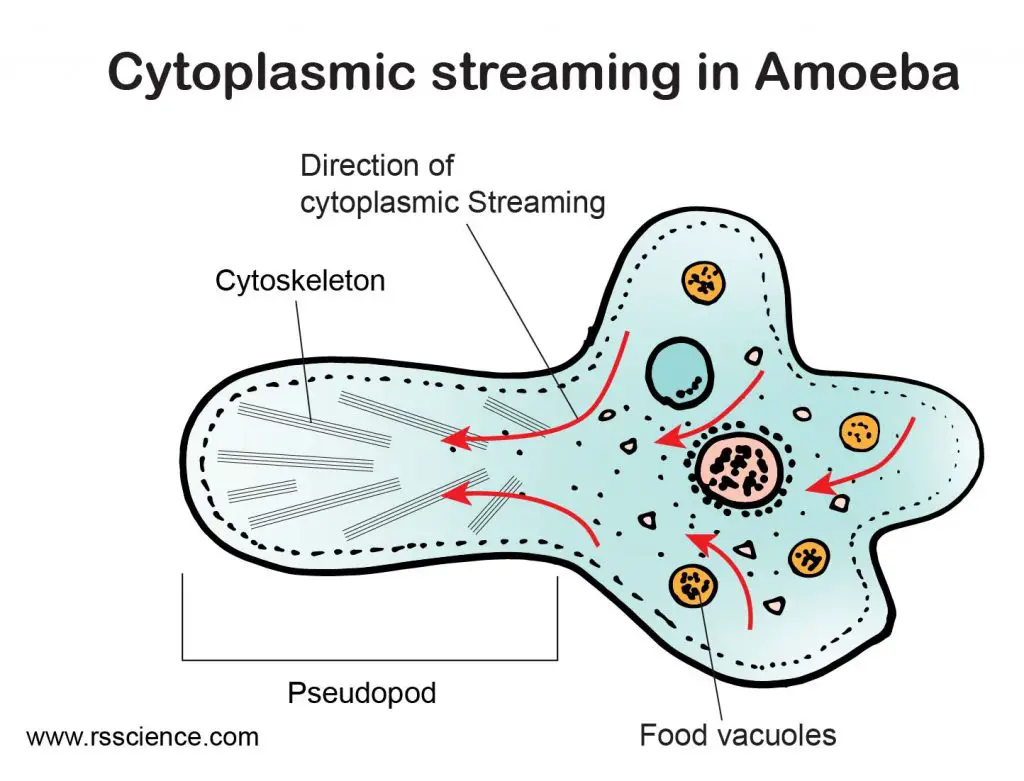
[In this figure] Cytoplasmic streaming in Amoeba.
Cytoplasmic streaming brings the cytoplasm and organelles to the new space after the movement of pseudopods.
Chara
Multicellular alga, Chara, need cytoplasmic streaming to distribute nutrients throughout their long cells. Their cells can grow up to 10 cm long and 1 mm in diameter. The cytoplasmic streaming circulates around a big central vacuole. The flow speed of Chara’s cytoplasmic streaming can reach a rate of 100 µm/sec, the fastest of all known cytoplasmic streaming phenomena. Chara is the most studied model organism for cytoplasmic streaming.
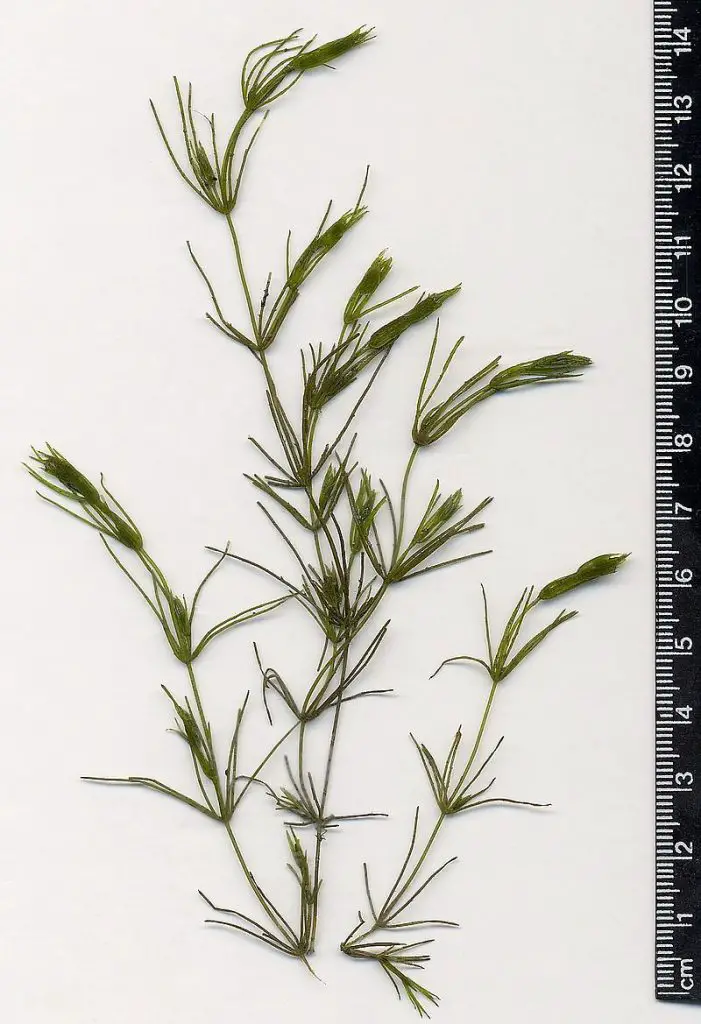
[In this figure] The green alga, Chara.
2. Increased efficiency of photosynthesis in plant cells
Cytoplasmic streaming also plays an important role in the photosynthesis of plant cells. Photosynthesis converts light energy into chemical energy. This occurs in the chloroplasts of plant cells.
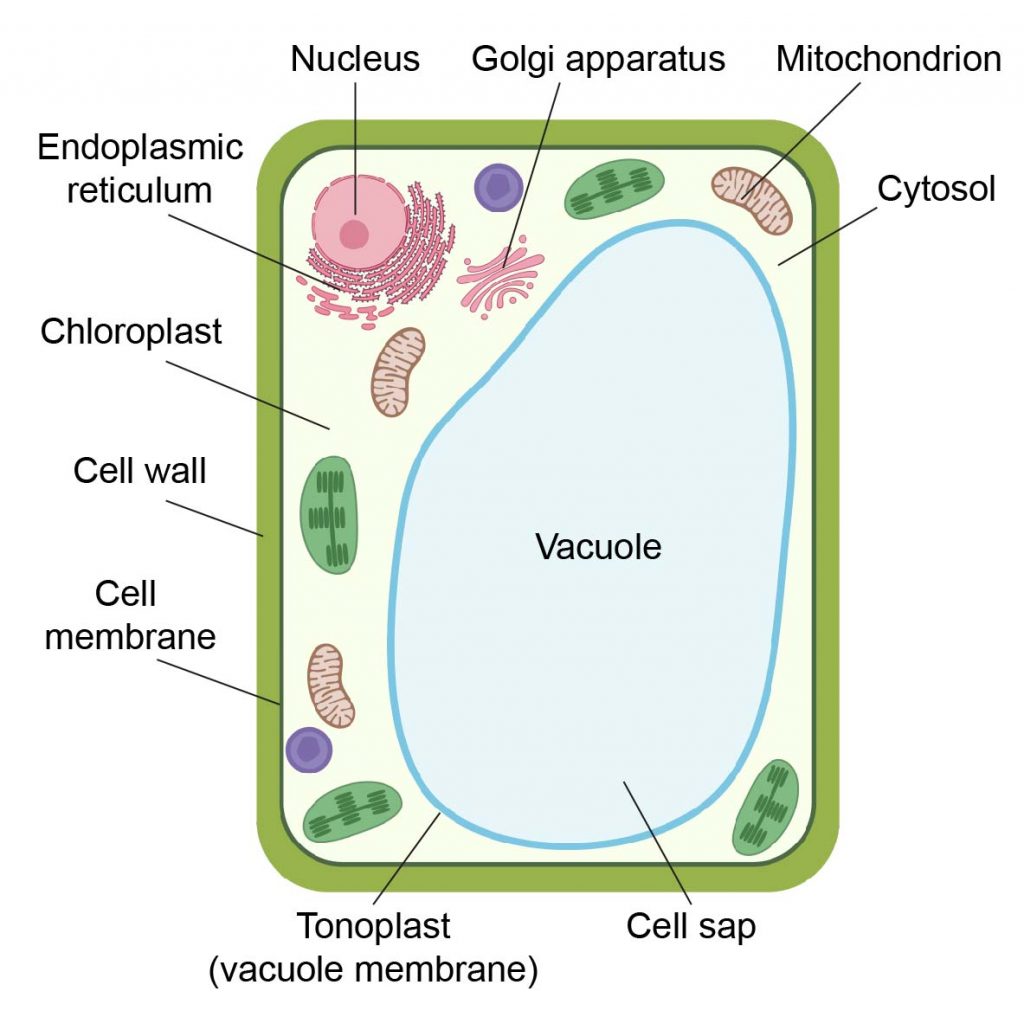
[In this figure] Typical structure of a plant cell.
Created with BioRender.com
Chlorophyll is the green molecule that absorbs the energy from Sunlight. However, if the light keeps illuminating the same chloroplasts and their chlorophyll molecules, they will become saturated with photons, making them unable to function until the saturation is alleviated (known as the Kautsky effect).
Chloroplasts need to be moved around to a position of optimum light absorption. Thus, the chloroplasts move into lighted regions and shaded regions, alternately. This intermittent exposure to light actually increases the photosynthetic efficiency of chloroplasts. The rate of motion is usually affected by light exposure, temperature, and pH levels. For example, during the evening or winter, the cytoplasmic streaming will slow down.
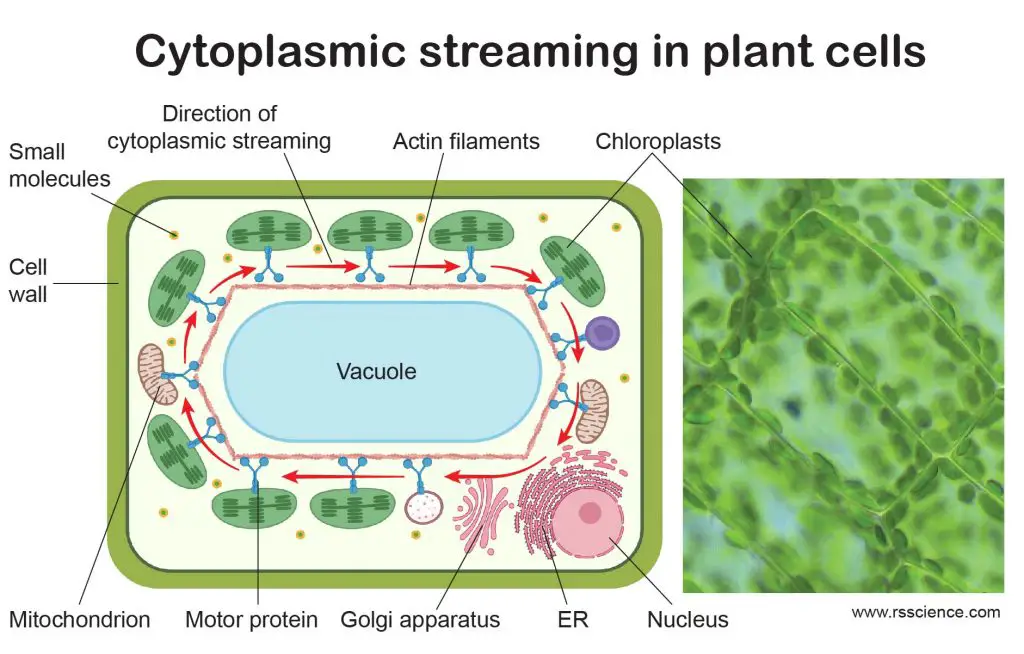
[In this figure] Cytoplasmic streaming in plant cells.
Cytoplasmic streaming circulates the chloroplasts around the central vacuoles in plant cells. This optimizes the exposure of light on every single chloroplast evenly, which can maximize the efficiency of photosynthesis. The right image is the actual cytoplasmic streaming of chloroplasts in Elodea cells.
Created with BioRender.com
Here is a very interesting study of cytoplasmic streaming in plant cells. Scientists compared different kinds of motor proteins (called myosin XI) in Arabidopsis (a widely studied model organism of plants). They found the movement rates of motor protein can be tuned by genetically exchanging its “feet”.
For example, using the “feet” from Chara alga can generate a high-speed motor protein (moves in 16 µm/sec), and using the human cell’s “feet” will result in a low-speed version (0.2 µm/sec). More interesting, the plants which possess “high-speed” motor proteins have a more efficient cytoplasmic streaming and can grow bigger. On the other hand, the plants with “low-speed” motor proteins are much smaller in size, suggesting that cytoplasmic streaming may affect the growth of plant cells.
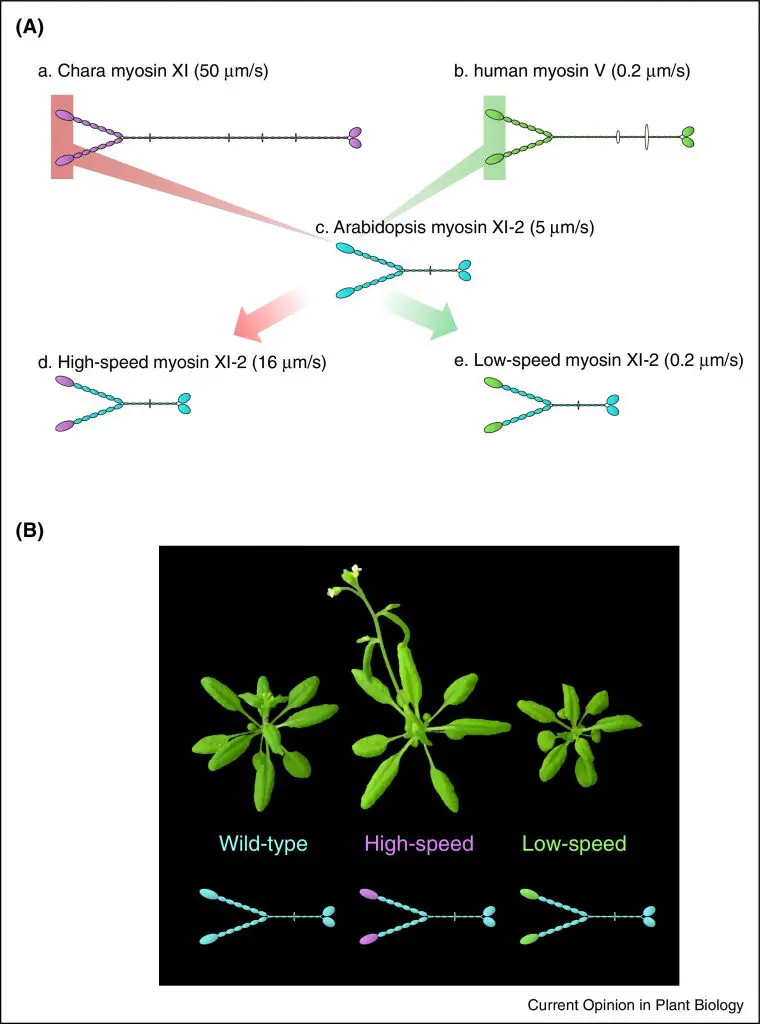
[In this figure] The high-speed and low-speed motor proteins cause different cytoplasmic streaming in plant cells, resulting in a dramatic difference in the size of plant growth.
Source: Current Opinion in Plant Biology.
3. Allow efficient transportation of nutrients across cells in plants and fungi
Structurally, plants and fungi have several common characteristics. They both have cell walls around their cells. They also have long tube-like cellular structures to build the foundation of organisms. In plants, xylem and phloem, both are tubular channels made of special cells, form the vascular system as the core of plant’s stems and leaves. In fungi, cells grow like filaments, called hyphae, into a network.
Fungus hyphae can be divided into individual cells by partitions called septa (singular: septum). Septa are specialized cell walls that consist of many tiny pores. Cytoplasmic streaming can transport molecules through these pores across cells. This allows an efficient allocation of nutrients in multicellular fungi (like molds and mushrooms).
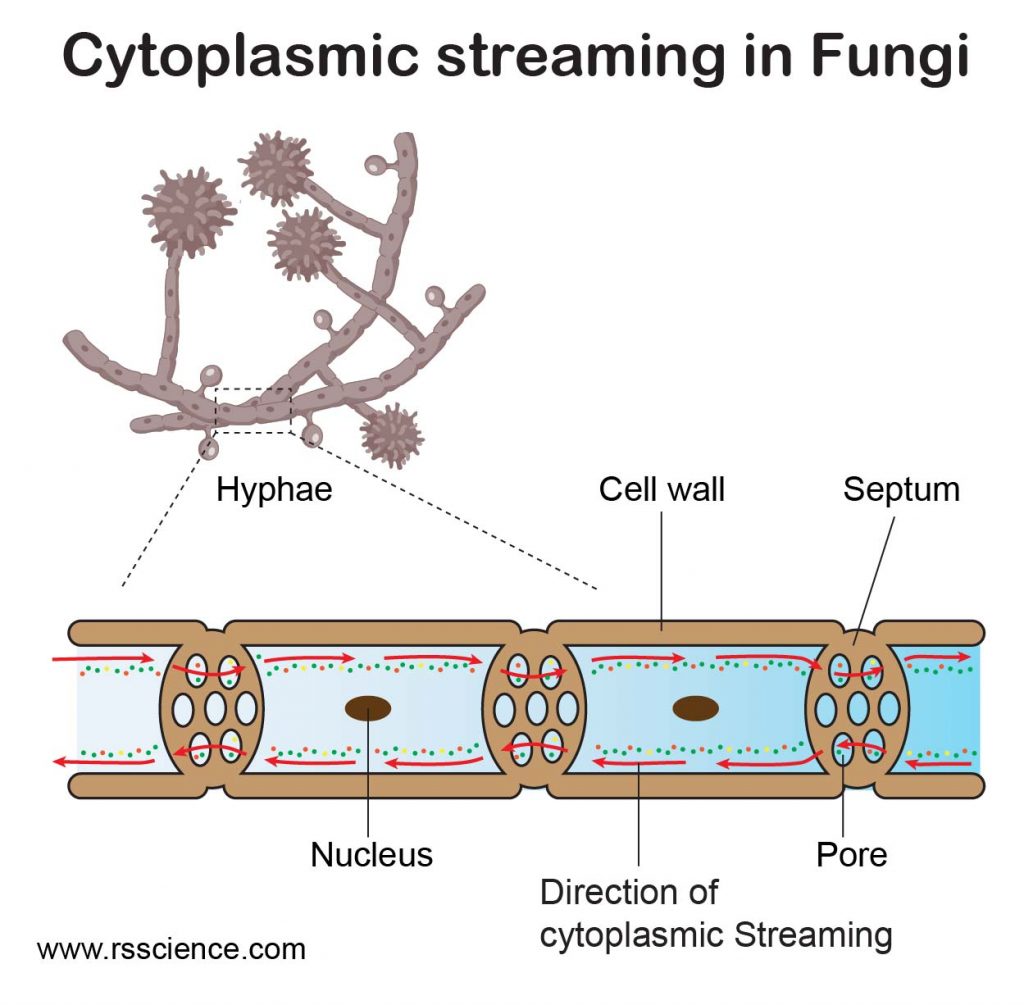
[In this figure] Cytoplasmic streaming in fungi.
Cytoplasmic Streaming brings nutrients to flow between cells through small pores.
Created with BioRender.com
[In this video] Cytoplasmic streaming functions as Fungal Freeways.
In vascular plants (including ferns, trees, and all flowering plants; not including mosses, liverworts, and hornworts), there is a vascular system that includes xylem and phloem. Xylem transports water and minerals from roots to shoots and leaves unidirectionally. The driving force in the xylem is the negative pressure due to the water loss from the leaves.
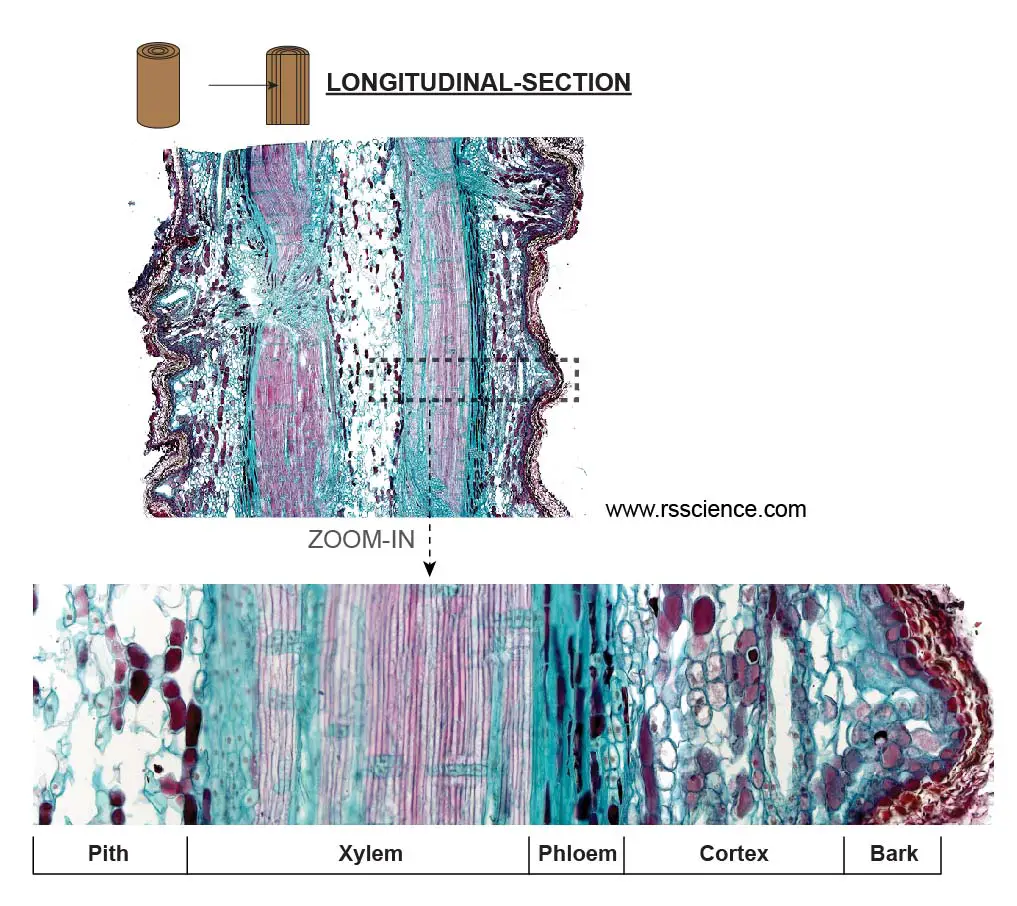
[In this figure] Microscopic image of a pine stem (longitudinal section) showing the key components of the plant’s vascular system. You can find this prepared slide here.
Phloem transports the organic molecules between parts of the plants bidirectionally. From leaves to roots, gravity can drive the movement of organic molecules. However, in order to deliver nutrients, like sugar, from lower to higher parts, phloem needs to spend energy and uses cytoplasmic streaming to achieve this job.
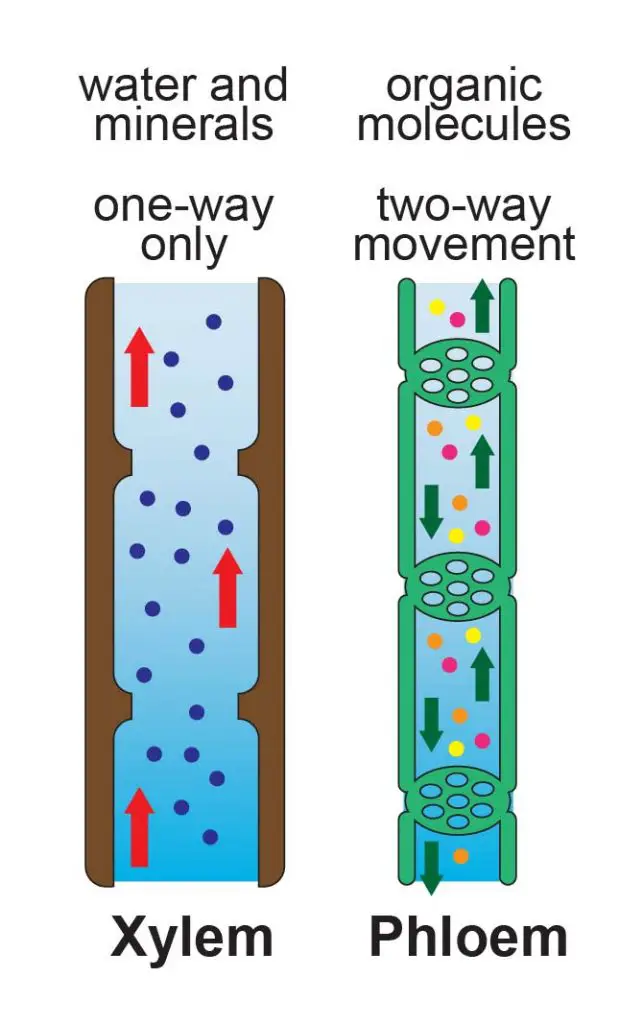
[In this figure] Xylem and phloem.
Xylem and phloem are both transport vessels that combine to form a vascular bundle in higher order plants. Organic molecules (like sugars produced by photosynthesis) can travel up or down in phloem. Phloem is made up of connected Sieve tubes. Between two Sieve tubes, there is a porous Sieve plate. Cytoplasmic streaming can bring molecules to flow through these small pores and move upward along the phloem.
4. Align the mitotic spindles in mammalian oocytes
Like we mentioned before, most human cells are relatively small and do not rely on cytoplasmic streaming. However, an exception is the oocytes (immature egg cell).
Cytoplasmic streaming plays a very special role in mouse oocytes – to keep the nuclei of the oocytes at a central position during division. In normal cells, centrioles and spindles keep nuclei centered within a cell for both mitosis and meiosis. Without such a centering mechanism, disease and death can happen. While mouse oocytes do have centrioles, they play no role in nucleus positioning, yet. The nucleus of the oocyte still maintains a central position due to cytoplasmic streaming. Although scientists found this phenomenon in mouse oocytes, they believed that it is a common mechanism in all mammalians, including humans.
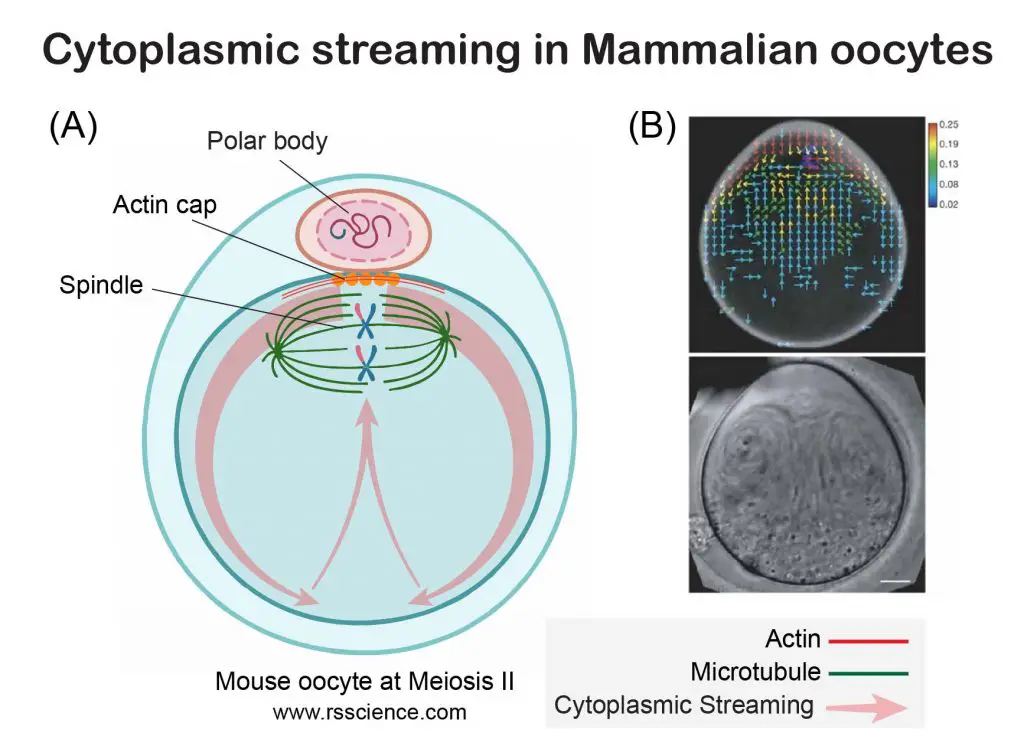
[In this figure] Cytoplasmic streaming in the mouse oocyte.
A study showing the special cytoplasmic streaming flow pattern in the mouse oocyte can maintain the dividing spindle in a central position.
[In this video] A video showing the cytoplasmic streaming in a mouse oocyte. The blue dots in the left video indicate the chromosomes.
Does cytoplasmic streaming happen in human cells?
For most mammalian and human cells, cytoplasmic streaming does not happen (oocyte is an exception). This is because our cells are relatively small ~20-50 µm in diameter compared to large single-celled organisms (such as Paramecium is 200-300 µm). In this case, diffusion is sufficient for small molecule distribution. However, our cells still have motor proteins to move organelles around and deliver specific molecules/proteins to certain subcellular locations.
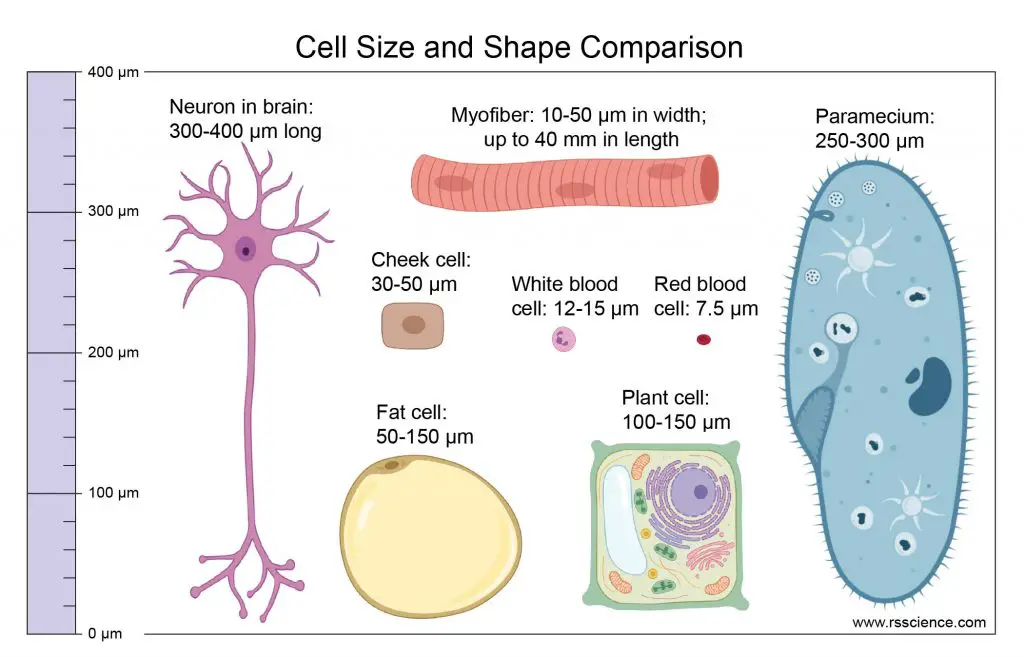
[In this figure] Different cell sizes and shapes comparison.
Most human cells are relatively small compared to paramecia and plant cells. Some cells (like neurons and muscle cells) may be pretty long. However, they are also pretty thin as well. Even a big fat cell has most of its cytoplasm very close to the cell membrane (the center is occupied by a huge oil droplet). Therefore, cytoplasmic streaming is not notable in most human cells. On the other hand, plant cells and single-celled organisms (like paramecium or amoeba) are larger in size and require cytoplasmic streaming to distribute substances in these cells.
Note: µm = micrometer = 1/1,000,000 meter.
Created with BioRender.com
How cytoplasmic streaming work?
The mechanism of cytoplasmic streaming is not completely understood. Currently, scientists believe the cytoplasmic streaming is mediated by “motor” proteins in the cells. As the name suggests, “motor” proteins can move along the cytoplasm of cells. These motor proteins “walk” along a molecular catwalk, which is made of filament proteins called cytoskeletons. Using this catwalk, the motor proteins can carry loads several times bigger than their size from one site to another.
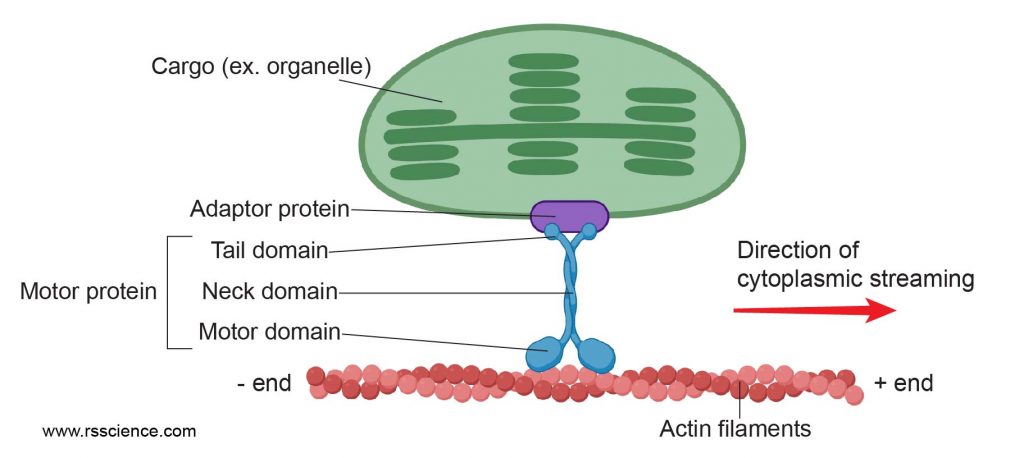
[In this figure] Motor protein walks on a molecular catwalk made of cytoskeletons (here, actin filaments) to deliver a cargo (for example, a chloroplast). This movement also generates a flow of cytosol, resulting in cytoplasmic streaming.
Created with BioRender.com
Motor proteins
Motor proteins have two “hands” and two “feet”. The hands and feet are both “sticky” – like post-it-notes. Just as a human can walk by placing one foot in front of the other, the motor proteins “walk” by swinging one foot in front of the other. Motor proteins walk along with the filament proteins of cytoskeletons, much like a trapeze artist walking on a high wire. At the same time, motor proteins can carry cargos using their hands. The sticky patches on the “hands” prevent the cargo from falling off as the motor proteins set off on their journey.
[In this video] A carton showing the action of motor proteins.
Motor proteins use adenosine triphosphate (ATP) as the energy currency to move. Each step uses one molecule of energy. The motor proteins need 125,000 steps to move 1mm along the cytoskeletons – that is a lot of energy!😅
When motor proteins drag organelles to move, the motion also creates a current surrounding the cytosol. If many motor proteins move along one direction (for example, move clockwise around the central vacuole in a plant cell), many these small flows join and become powerful cytoplasmic streaming. Dragging endoplasmic reticulum (ER) is the most efficient way to generate a cytoplasmic streaming. This is because that ER is a web-like structure with a significant surface area interacting with the cytosol. You can imagine that there are many fishing boats trawling together inside a small cell.
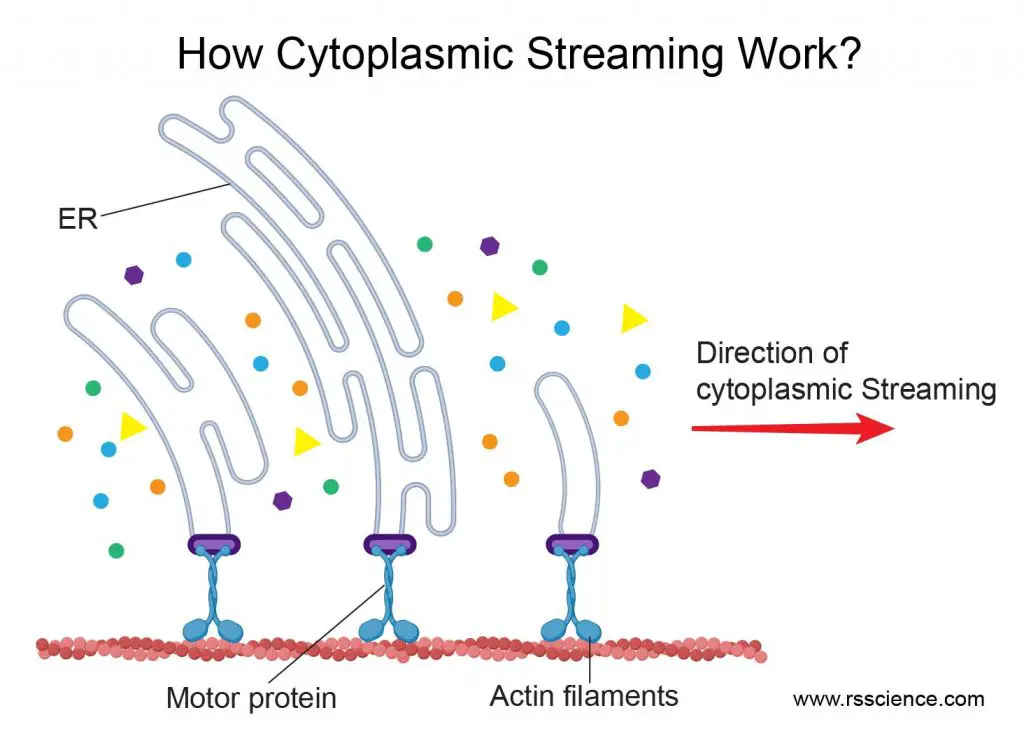
[In this figure] Motor proteins dragging the movement of ER webs can efficiently generate a cytoplasmic streaming in the cell.
Created with BioRender.com
What affects cytoplasmic streaming?
Changes in physiological conditions of the cells can affect the speed, direction, and pattern of cytoplasmic streaming. Scientists had found these conditions:
- Temperature
- pH value
- Ion concentrations – especially chloride, magnesium, and calcium
- Viscosity of cytoplasm
- Hormone
- Light exposure
Chemicals that interact with cytoskeletons may also affect cytoplasmic streaming. For example, Cytochalasin D is a toxin that can disrupt actin filaments and stop cytoplasmic streaming.
The discovery of cytoplasmic streaming
Cytoplasmic streaming was first reported in 1774 by an Italian physicist Bonaventura Corti, who found the flow of cytoplasm when he observed cells of algae Nitella and Chara. The presence of cytoplasmic streaming helped convince biologists that cells are the fundamental units of life.
More cytoplasmic streaming in videos!
Finally, let’s look at more cytoplasmic streaming in different types of cells.
[In this video] Cytoplasmic streaming in onion skin cells.
[In this video] Cytoplasmic streaming in slime molds.
[In this video] Cytoplasmic streaming in a pollen tube.
[In this video] Cytoplasmic streaming in Spirogyra (a green, filament algae).
[In this video] Cytoplasmic Streaming in Eelgrass (Vallisneria americana).
[In this video] Cytoplasmic streaming in Raphidiophrys.
Raphidiophrys elegans always like to form colonies with neighboring cells. They connected by special cytoplasmic bridges (tube structure between cells). Cytoplasmic streaming allowing the exchange of organelles (granules) along cytoplasmic bridges from one cell to another. This possibly serves as a system to keep the colony level.
[In this video] Cytoplasmic streaming in Diatoms.
[In this video] Cytoplasmic streaming in Tradescantia flower’s stamen hair cells.
Reference
“History of Cell Biology” – Ariane Dröscher
“Cytoplasmic streaming” – Wikipedia
Pingback: What is the function of cytoplasmic streaming? – Dr. Biology Questions and Answers