In this section, we will go through the cell structure of paramecium. Unless mentioned, we refer to Paramecium caudatum as a typical example of a paramecium cell.
We also have 4 series of blog posts about paramecium:
Part I. The Biological Classification of Paramecium – Name, History, and Evolution
Part II. The Structure of Paramecium cell
Part III. Paramecium Reproduction, Physiology, and Behaviors
Part IV. The Natural Habitation and Cultivation of Paramecium – Find Paramecium for Your Microscopic Project
This article covers
The anatomy of paramecium
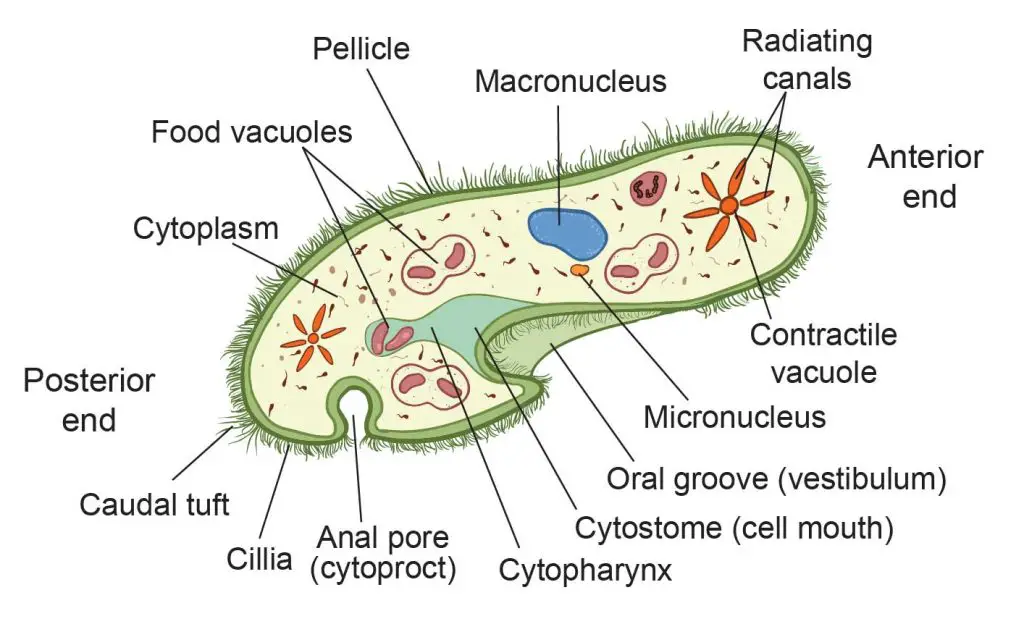
[In this figure] The labeled diagram showing the anatomy of a Paramecium cell.
Each structure/organelle and its function will be explained in this article.
Paramecium wears a soft armor, called pellicle
The body of the paramecium cell is enclosed by a stiff but elastic membrane, called pellicle. Pellicle is made up of a thin, gelatinous substance produced by the cell. The layer of the pellicle gives the paramecium a definite shape and good protection of its cell content. The pellicle is also elastic in nature which allows the paramecium to slightly change its shape.
Paramecium’s skin is covered by many tiny hairs, called cilia
Covering the pellicle are many tiny hairs, called cilia (singular cilium). Cilia are projections distributed throughout the cell. One P. caudatum cell can have 5000–6000 cilia.
Cilia are very tiny structures – approximately 0.25 μm in diameter and up to 20 μm in length. We can only see the overall motion of cilia on the cells but hard to see them clearly under a regular light microscope.
[In this video] Paramecium cilia movement under a microscope.
There are two types of cilia present on the cells of paramecia: oral cilia and body cilia. Oral cilia are present on the surface of the oral groove. They help collect food materials. Body cilia are on the body surface and facilitate its locomotion. They act like microscopic oars to move the organism in one direction.
Body cilia are arranged in longitudinal rows (along the head-to-tail axis) with a fairly uniform length throughout the cell. There are also a few longer cilia present at the posterior end of the cell (quite obvious in P. caudatum). These form caudal tuft of cilia (hence the name caudatum).
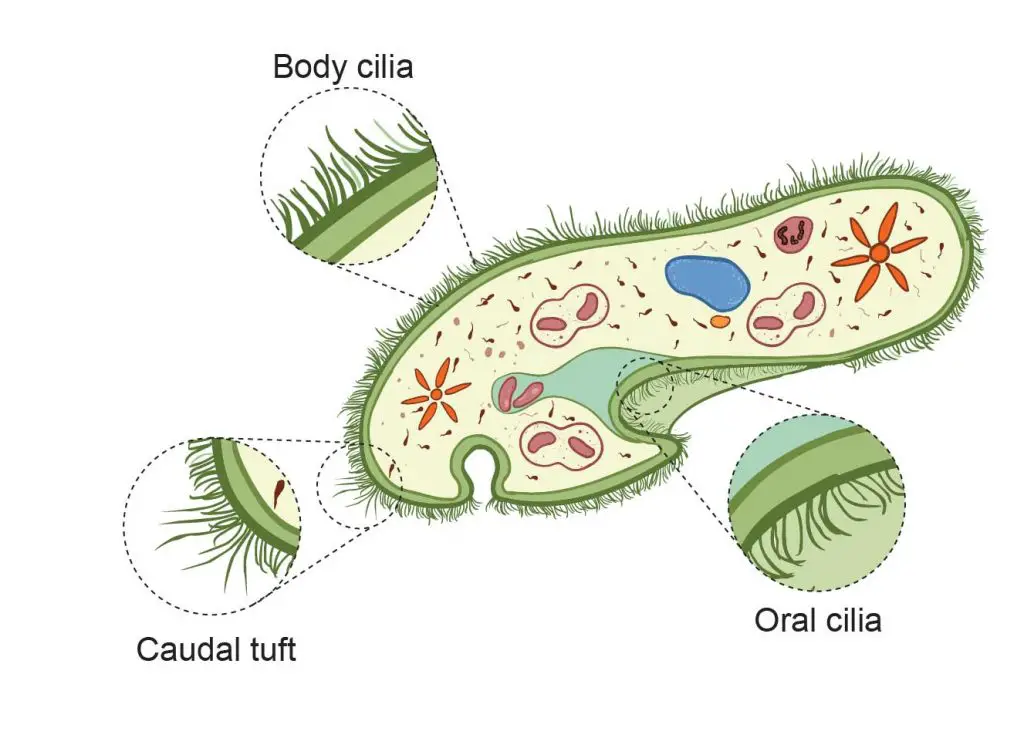
[In this figure] Different types of cilia on paramecium cell – oral cilia, body cilia, and caudal tuft.
The microscopic view of cilia
Scientists spent a lot of time and effort studying paramecium cilia. Why?
It is because cilia are not exclusive in microorganisms, like paramecia or ciliates. In fact, we also have cilia on our cells. For example, motile cilia are found on the respiratory epithelium lining the respiratory tract where they clean our lungs by sweeping mucus and dirt out.
Advanced microscopy is powerful in these kinds of cell biology research. For example, the scanning electron microscope (SEM) allows us to see the morphology, orientation, and density of paramecium’s cilia. With a transmission electron microscope (TEM), we can see the ultrastructure of cilia in a transverse section. With the help of antibody-based immunofluorescent staining, scientists can even see what kinds of proteins contribute to the structure, motion, and growth of cilia.
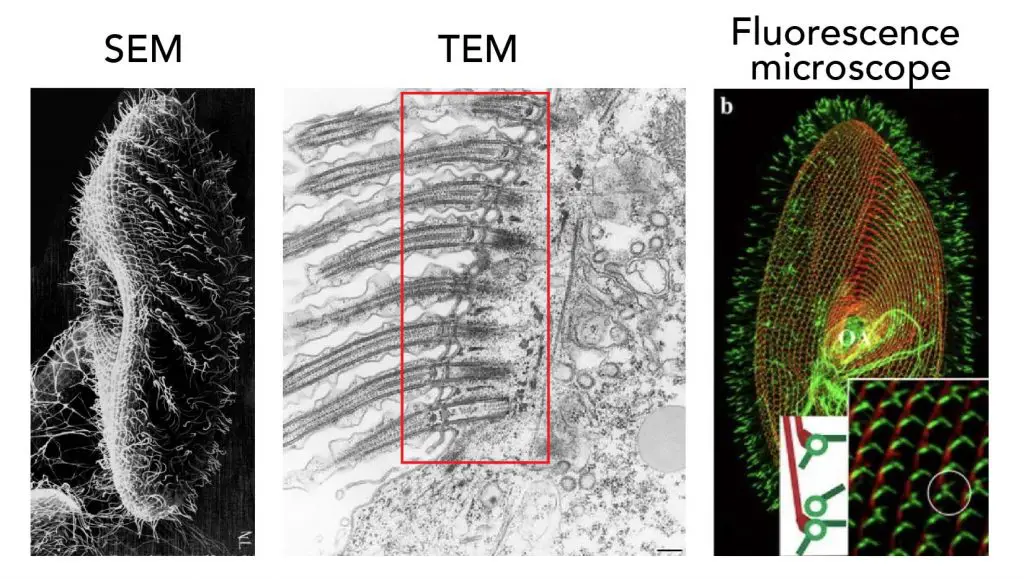
[In this figure] Scientists used advanced microscopes to answer their questions of paramecium’s cilia. Left: SEM shows us the morphology of cilia (Credit: Judith L. Van Houten). Middle: TEM gives us the transverse section image of cilia in detail (Credit: Richard Allen). Right: Fluorescence microscope shows us how cilia anchor on the cell’s surface.
The structure of pellicle and cilia
With the help of advanced microscopy, scientists now know how the cilia grow and move in detail. As you can see in the illustration below, the layer of pellicle is not smooth. Instead, there are many bumps (called alveoli) with a depression on the pellicle. A cilium comes out through the center hole of each depression with the anchor on the basal body.
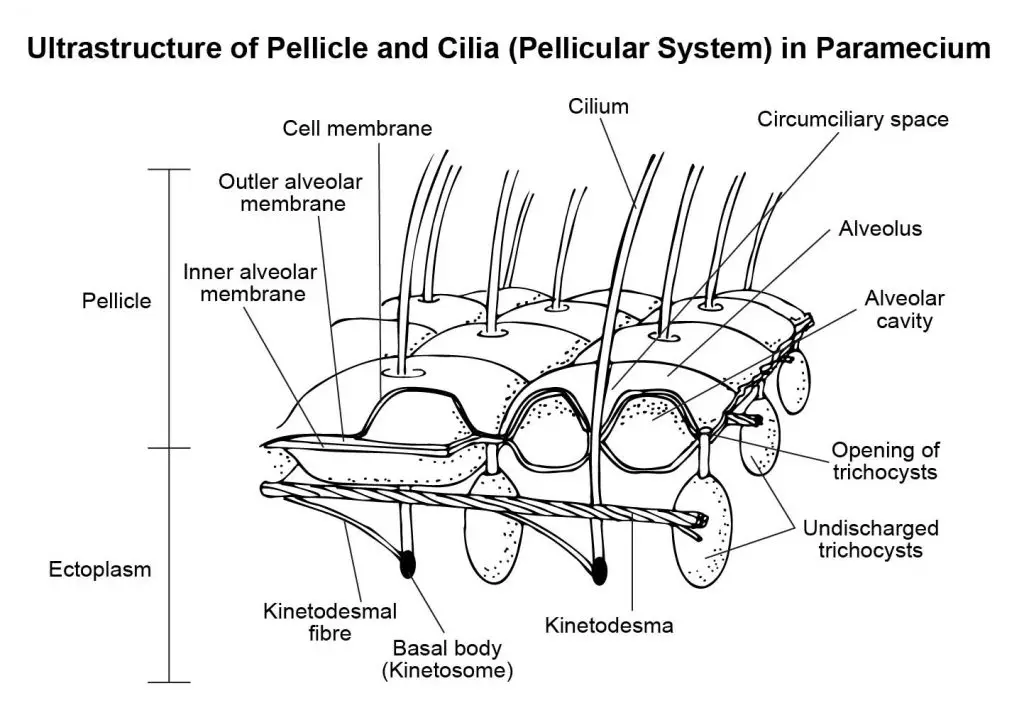
[In this figure] The detailed structure of cilia and pellicle.
Scientists also discovered what is inside each cilium hair. A cilium is made up of microtubule bundles. Microtubules are protein fibers inside the cells with multiple functions. Microtubules can serve as an intercellular highway for the transportation of molecules and organelles. During cell division, microtubule fibers projected from two centrosomes pull chromosomes apart into new nuclei.
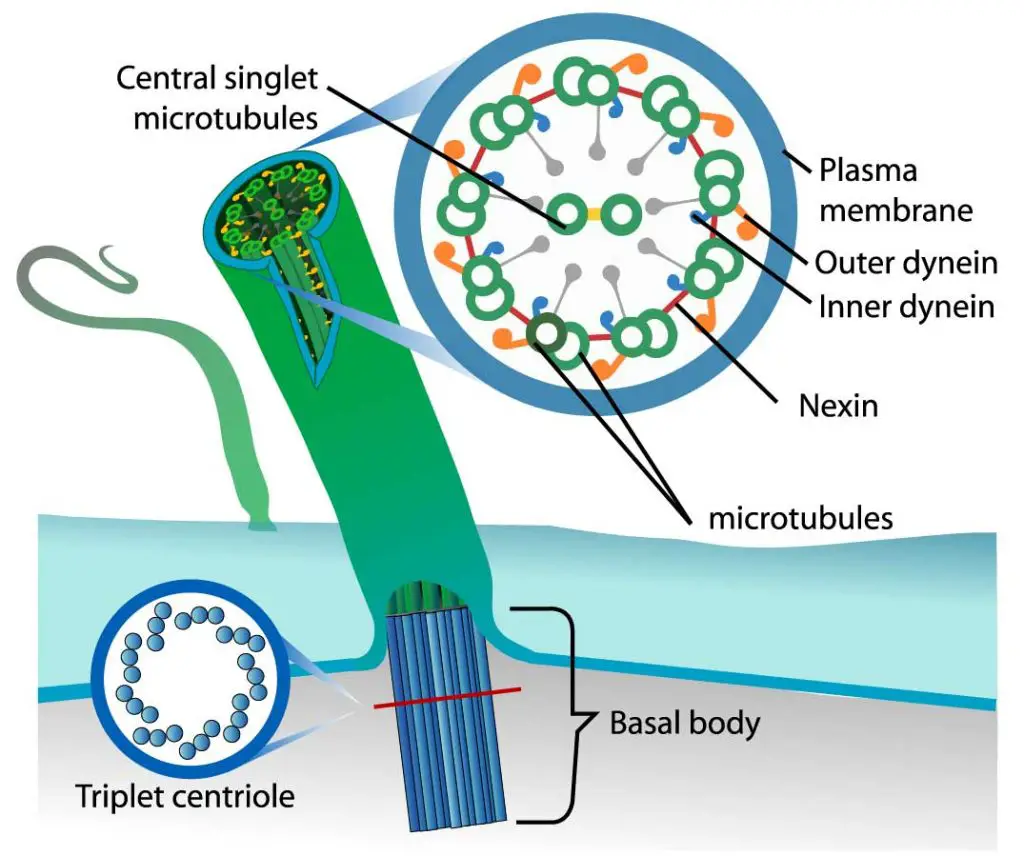
[In this figure] The organization of cilium.
Each cilium contains nine pairs of microtubules forming the outside of a ring and two central microtubules. This structure is known as an axoneme. Microtubules are held together by cross-linking proteins. There are motor proteins, called dynein, sitting across each paired microtubule fiber.
Photo credit: LadyofHats on wiki.
The motor proteins (dynein) use ATP as energy to crawl along the microtubules. When dynein proteins move upward on one side but downward on the other side, the cilium bends. The repeat of bending-relaxing cycles makes cilia act like oars, beating back and forth to create movement.
See how cilia do the wave
If the cilia just wave back and forth in the same way, the cells can’t go anywhere. The forward and backward strokes have to be in different phases to create a meaningful propulsive force.
Scientists used a microscope with a high-speed video camera to capture how cilia beat to propel the entire body of paramecium. They look pretty smart!
[In this video] Motion of cilia exhibits beautiful metachronal wave-like coordination where a constant phase difference is maintained between adjacent cilia.
By analyzing the high-speed video frame by frame, scientists found that the paramecium swims in a way similar to how we swim in the front crawl stroke.
Effective (forward) stroke: During the effective stroke, the cilium extends straight up (in order to engage more water) and beats against water, thus bringing the body forward and sending the water backward.
Recovery (backward) stroke: During recovery stroke, the cilium comes back to the original position by its backward movement. The cilium tends to bend and stay closer to the cell surface to minimize the resistance.
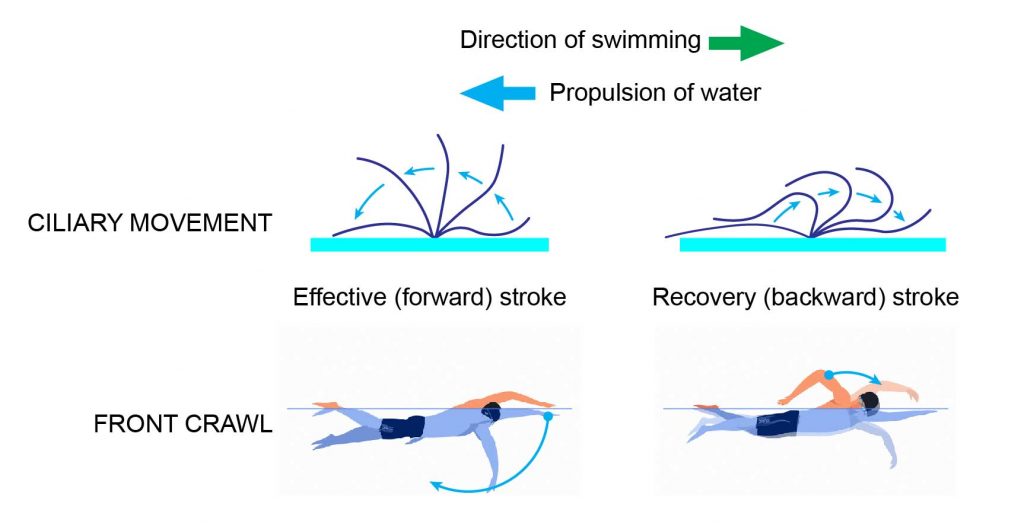
[In this figure] Stroke pattern of cilia on a Paramecium.
The movement of cilia can be divided into Effective (forward) and Recovery (backward) strokes. Two kinds of strokes alternately repeat to propel the body of paramecium as we swim in the front crawl style.
Unlike us that only have two arms, a paramecium cell has thousands of cilia. In order to swim efficiently, all the cilia do not move at a time. Cilia group into two types of coordinated rhythms.
Synchronous rhythm – Cilia of transverse row move at the same time.
Metachronous rhythm – Cilia of longitudinal row beat one after another. This creates metachronal waves passing from the anterior to the posterior end.
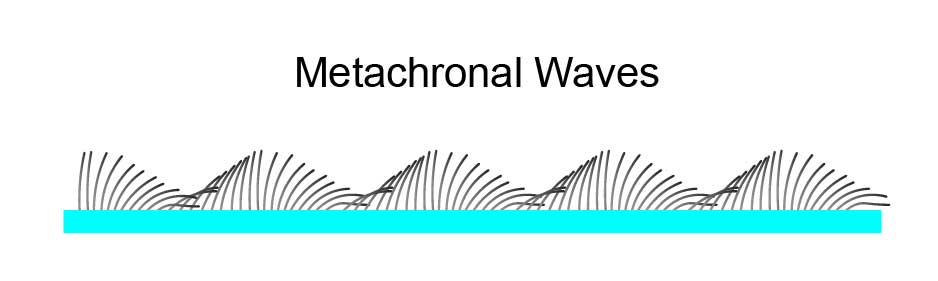
[In this figure] Cilia move in Metachronous rhythm to create a propulsive force.
Another interesting behavior is paramecium’s way of escape. If a paramecium comes across an obstacle, the beating of the cilia stops and reverses. This causes the paramecium to swim backward to keep away from the obstacle or the predators.
How fast can a paramecium move?
You may wonder how fast the paramecium can move? They move faster than Olympic gold medalists!
Most ciliates like the paramecia are incredible swimmers. Why cilia? When you are less than a millimeter in body size, water is like sticky syrup. Swimming like a fish would not be very efficient! If you want to swim fast and be able to maneuver, cilia are the best choice.
The cilia of paramecium move like many tiny oars, propelling the organism through the water at a rate that is “four times its body length per second”. For a P. caudatum which is 300 micrometers (µm) in length, it can swim at a rate of 1200 µm per second (equal to 0.0027 miles per hour). If Michael Phelps (6 ft 4 in or 1.93 m) swims like a paramecium, he will swim at a rate of 7.72 meters per second and finish a 100-meter course in 12.95 seconds. That is four times faster than the world record in swimming!
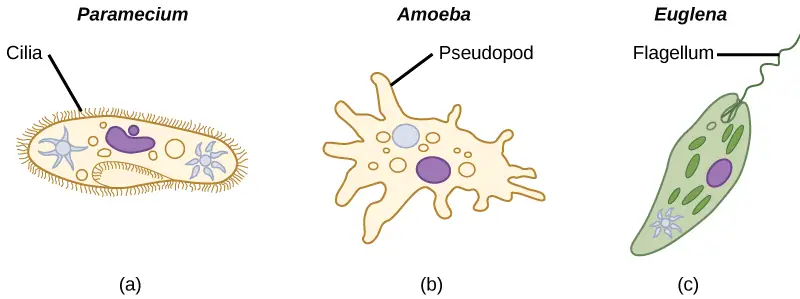
[In this figure] Three ways of locomotion for protozoa.
Cilia – coordinately beat to swim. Pseudopod – crawl on the surface by changing the cell shape. Flagellum – swim by rotating like a propeller.
Photo credit: Lumen.
How does a paramecium eat?
Oral Groove
Paramecia eat other microorganisms like bacteria, yeast, or algae. They eat through a system that works similarly to our mouth-esophagus-stomach. There is a large oblique shallow depression, called oral groove, on the ventro-lateral side of the paramecium’s body. This oral groove gives an asymmetrical appearance to the animal.
The oral groove serves as the entrance of food materials into the cell. There are oral cilia covering the surface of the oral groove. These oral cilia beat to create an inbound water current and bring the food into the oral groove.
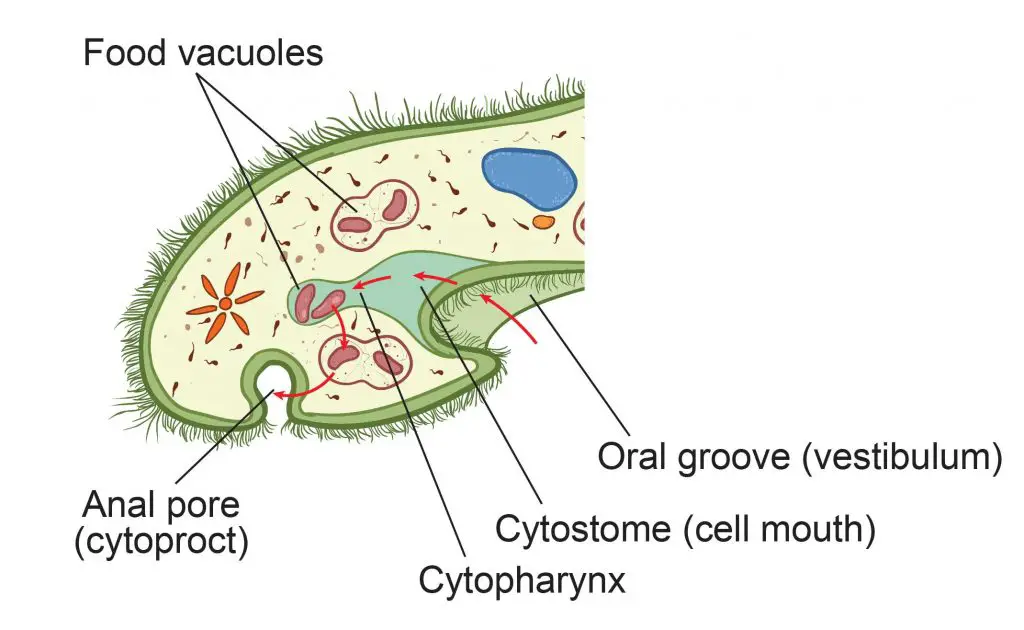
[In this figure] The closer view of paramecium’s feeding system.
You can follow the red arrows to track the path of food going through the paramecium’s feeding system. First, food particles are collected into the oral groove by the movement of the oral cilia. The food materials travel from cytostome to cytopharynx, and then into food vacuoles by phagocytosis. Digestive enzymes inside the food vacuoles break down the food into small nutrient molecules. After nutrients are absorbed into the cytoplasm by the cell, the indigestible debris is discharged from the anal pore.
Cytostome, Cytopharynx, and Food Vacuole
The end of the oral groove connects to a funnel-like structure, called cytostome or cell mouth. Oral cilia also cover the lumen of cytostome to bring the food particle down to the bottom of the cytostome funnel, which extends into the cytopharynx.
The cytopharynx is a tube-like structure (like our esophagus) that leads to the food vacuoles. Food vacuoles form by budding from the posterior end of cytopharynx. Food vacuoles function like our stomach and contain digesting enzymes to break down the food materials into nutrient molecules.
The feeding process by engulfing through food vacuoles, called phagocytosis, is common in single-celled microorganisms such as Amoeba. However, only the members of the ciliates and Euglena families develop the special cytostome-cytopharynx system.
Does a paramecium make a poo?
Anal Pore
The answer is Yes. Paramecia have their way of excretion. After the nutrients from digested food have been absorbed into the cytoplasm, there is still indigestible debris inside the food vacuoles. The waste will be ejected from a structure called the anal pore or cytoproct.
Various single-celled eukaryotes have the anal pore. The anal pore of a paramecium is a region of the pellicle that is not covered by ridges and cilia. The thin pellicle allows vacuoles to be merged into the cell surface and emptied.
The specialized “Skin” of paramecium cell body
Ectoplasm and endoplasm
Unlike the multi-celled organisms that have a layer of dedicated skin cells as a protective barrier, single-celled paramecium develops a “cellular skin” to protect itself.
As we mentioned above, the outermost layer is the soft shell of pellicle and cilia. Bound to pellicle is a narrow peripheral layer of specialized firm cytoplasm, called the ectoplasm. Below the ectoplasm lies a more fluid type of cytoplasm: the endoplasm. This region contains the majority of cell components and organelles.
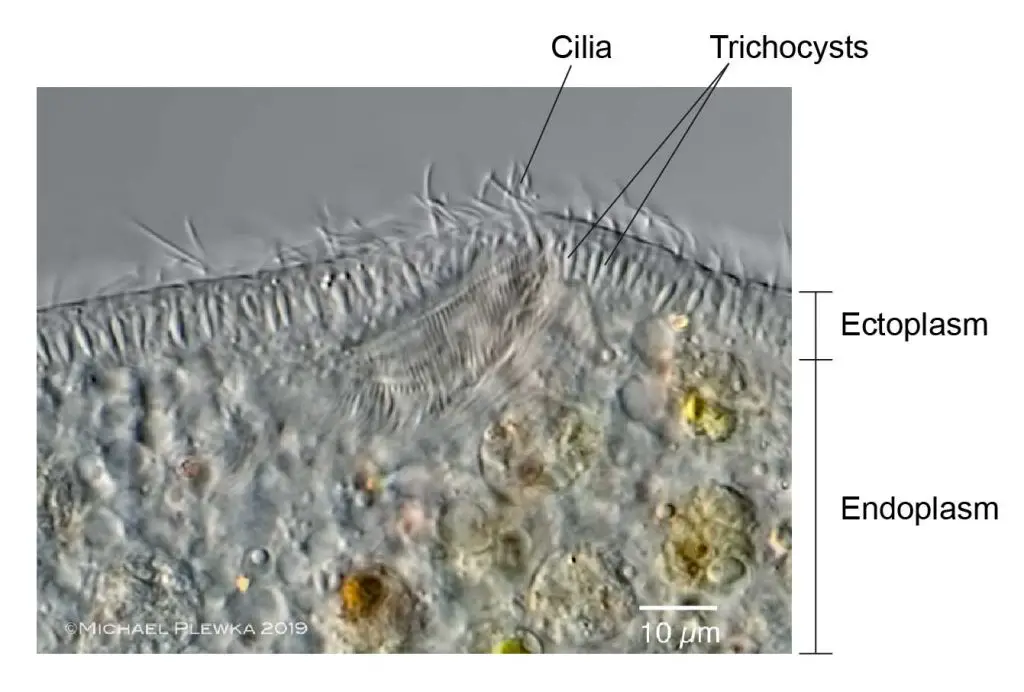
[In this figure] A closer view of the structure of paramecium’s skin.
In this high-resolution image of the paramecium cell, you can see two layers of cytoplasm: ectoplasm and endoplasm. Trichocysts are protective organelles embedded in the ectoplasm layer. (Image modified from Michael Plewka’s work)
Compared to the rest of the cytoplasm (endoplasm), ectoplasm forms a thin, dense, and clear outer layer containing trichocysts and fibrillar structures. The roots of cilia also anchor in the ectoplasm layer. Pellicle and ectoplasm together serve as the protective skin for paramecia.
Trichocyst
Trichocyst (trick-o-sists) is a small spindle-like organelle situated in the ectoplasm with a minute pore opened on the pellicle surface. Trichocysts are arranged perpendicular to the ectoplasm. Trichocysts are filled with a dense refractive fluid containing swelled substances. When the cells receive mechanical, chemical, or electric stimuli, trichocysts discharge their contents and become long, thin, stinging spikes. After they are discharged, new ones are generated from kinetosomes.
The exact function of trichocysts is not quite clear, though a popular theory is that they are important for defense against predators. Trichocysts may also help cell adhesion and support the paramecium cell body.
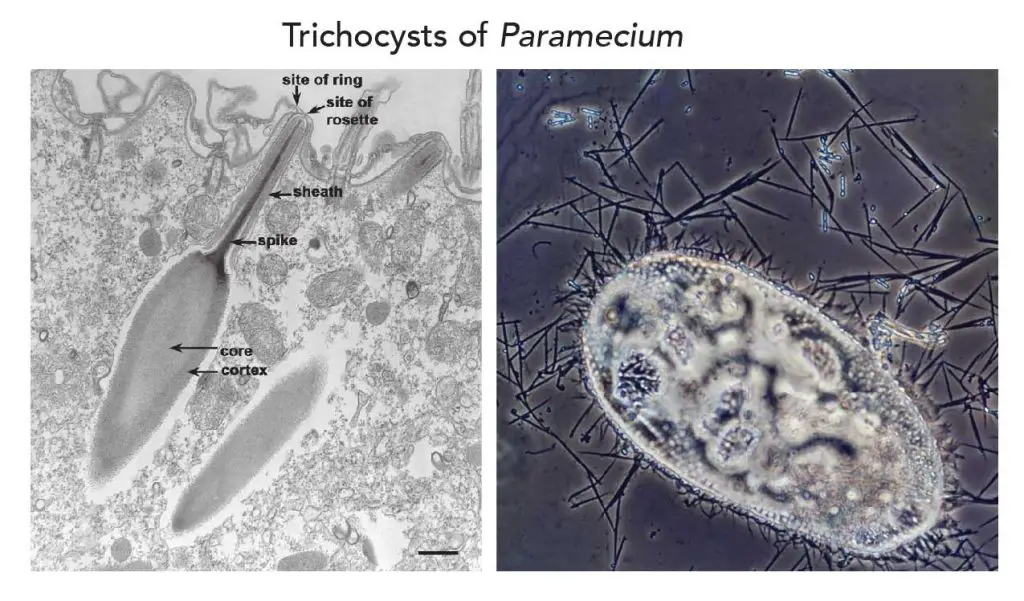
[In this figure] Trichocysts of Paramecium.
Trichocysts are spindle-like organelles that can discharge stinging filaments as a protection against predators. Left: A TEM image showing a trichocyst embedded in the ectoplasm. When receiving outside stimuli, the core of the trichocyst will swallow and push the spike out from the sheath. (Image: Bannister, J. Cell Sci. 11:899-929, 1972.) Right: Highly magnified phase contrast image showing a paramecium fired its spiky trichocysts for protection. (Image: Walter Dawn, Encyclopædia Britannica)
What is inside the cell body of a paramecium?
Cytoplasm and organelles
Like a normal eukaryotic cell, enclosed inside the pellicle layer of a paramecium is a jelly-like substance called cytoplasm. The cytoplasm includes the cytosol and all the organelles. The cytosol is like condensed soup inside the cell. It is a complex mixture of all kinds of substances dissolved in water. You can find small molecules like ions (sodium, potassium, or calcine), amino acids, nucleotides (the basic units of DNA), lipids, sugars, and large macromolecules such as proteins and RNAs.
A paramecium has all the common organelles of eukaryotic cells (link to Cell biology), including mitochondria (the cell’s powerhouses), endoplasmic reticulum and ribosomes (where protein synthesis), Golgi apparatus (the post office inside the cells), lysosomes (the storage of digestive enzymes), peroxisomes (the chemistry laboratory inside the cells).
Unlike plant cells, paramecium doesn’t have chloroplasts.
Unlike the regular eukaryotic cells, paramecium has two nuclei, a big one and a small one. Paramecium also consists of two types of vacuoles: contractile vacuole and food vacuole, which do not exist in human cells.
Paramecium is powered by a dual-core CPU – Macronucleus and Micronucleus
The most unusual characteristic of paramecia is their nuclei. They have two types of nuclei, which differ in their shape, content, and function.
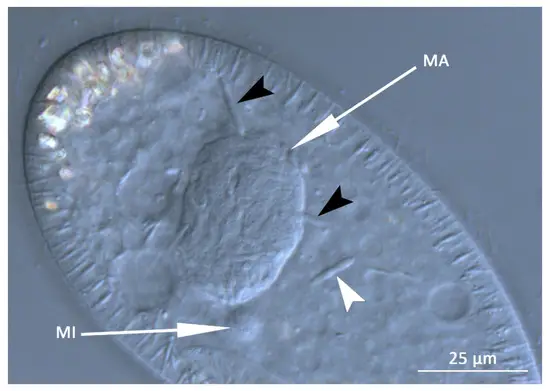
[In this figure] Macronucleus (MA) and Micronucleus (MI) in a P. putrinum cell. White and black arrowheads point symbiotic bacteria inside the cytoplasm.
Photo credit: MDPI
Micronucleus
The two types of nuclei are micronucleus and macronucleus. The micronucleus is diploid; that is, it contains two copies of each paramecium chromosome (human’s nucleus is also diploid). The micronucleus contains all of the DNA (called genome) that is present in the organism. This DNA is passed from one generation to another generation during reproduction.
Macronucleus
On the other hand, the macronucleus contains a subset of DNA from the micronucleus. These DNA fragments are copied from micronucleus to macronucleus because they carry genes that are frequently needed by the paramecium cell. Genes in the macronucleus are actively transcripted to mRNA and then translated to proteins. The macronucleus is polyploid or contains multiple copies of each chromosome, sometimes up to 800 copies.
The function of Micronucleus and Macronucleus
In other words, the function of the micronucleus is to maintain genetic stability and make sure that the desirable genes are passed to the next generation. It is also called the germline or generative nucleus. Macronucleus plays a role in non-reproductive cell functions including the expression of genes needed for the everyday function of the cell. The macronucleus is also called the vegetative nucleus.
If we use a computer as a metaphor, the micronucleus is the hard drive that keeps a complete copy of the cell’s program. The macronucleus acts as the random-access memory (RAM) which stores working data and machine codes. The computer only loads programs currently in use from hard drive to RAMs. In a paramecium cell, more active genes (meaning the cell need more of these proteins encoded by these genes) may have more copies in the macronucleus.
Another reason to have two distinct nuclei is that it is a mechanism by which paramecia and other ciliates can stave off genetic intruders (meaning pieces of DNA that spy themselves into the genome, for example, virus’ DNA).
By having two nuclei, if a piece of DNA is in the micronucleus but not in the macronucleus, it will be removed during the next round of cell division. In other words, if something foreign got into the micronuclear genome, then when the next macronucleus is made, it would be removed and not included in the expressed version [transcribed] of the genome. This mechanism functions as a primitive DNA immune system; that is, surveying the genome and trying to keep out invading elements.
Morphologically, the macronucleus is kidney-liked or ellipsoidal in shape. The micronucleus is found close to the macronucleus. It is a small and compact structure, spherical in shape. All paramecium species have one macronucleus. However, the number of micronuclei can vary by species. For example, P. aurelia has two micronuclei and P. multimicronucleatum has several.
Two kinds of vacuoles which are vital for paramecium
The name “vacuole” describes these organelles appear transparent and tend to be filled with fluid. Vacuoles take on specific functions in a paramecium cell. Paramecium has two types of vacuoles: contractile vacuoles and food vacuoles.
Contractile vacuoles act as water pumps for osmoregulation
One paramecium cell has two star-shaped contractile vacuoles sitting on each end of the body. They are filled with fluids and are present at fixed positions between the endoplasm and ectoplasm. Contractile vacuoles are responsible for osmoregulation, or the discharge of excess water from the cell.
How to deal with “osmosis” is a universal challenge for all living creatures. It is especially critical for unicellular microorganisms like paramecium.
As we know, each cell is surrounded by a cell membrane. This membrane has tiny holes that allow small molecules (like water) to pass through but not large ones (like salt). Due to this nature, the cell membrane is partially permeable. Osmosis is the movement of water molecules from an area of high water concentration (dilute solution) to an area of low water concentration (concentrated solution) through a partially permeable membrane.
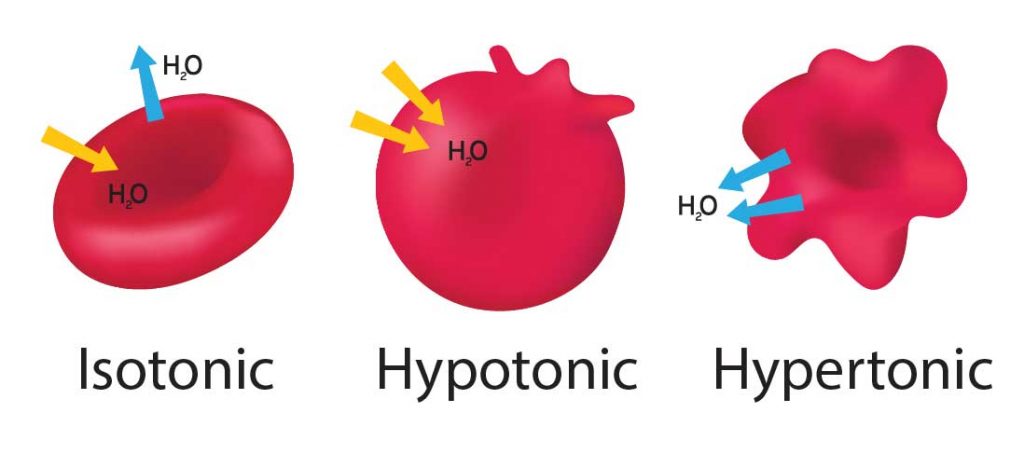
[In this figure] Animal cells (red blood cells as an example in this graph) are sensitive to osmosis pressure. When our cells are in an “Isotonic” environment (like our blood), the in and out of water molecules are equal, and the cells are safe. If the environment becomes “Hypotonic” meaning fewer solutes (minerals) than Isotonic, water will move into the cells to achieve balance. The cells will swell and even burst (lyse) if excess water is not removed from the cell. On the other hand, “Hypertonic” is due to more solutes in the environment and can cause cells to shrink.
The contractile vacuoles act to regulate the quantity of water inside a cell. In freshwater, which is a hypotonic environment for paramecium, water flows into the cell by osmosis. The contractile vacuoles expel water out of the cell by contracting and preventing the cell from absorbing too much water or even bursting.
How do contractile vacuoles work?
Each contractile vacuole is connected to several radical canals (which form its star shape). Excess water is drained from the whole body of paramecium and fed into the contractile vacuoles via these canals. The accumulation of water makes the vacuole increase in size. Once the reservoir reaches a contain threshold, the contractile vacuole contracts to discharge the excess water through a pore on the pellicle.
Two contractile vacuoles work independently. The posterior contractile vacuole is close to the cytopharynx and hence contract more quickly because of more water passing through. When the contractile vacuole collapses, it may disappear periodically and hence are called temporary organs.
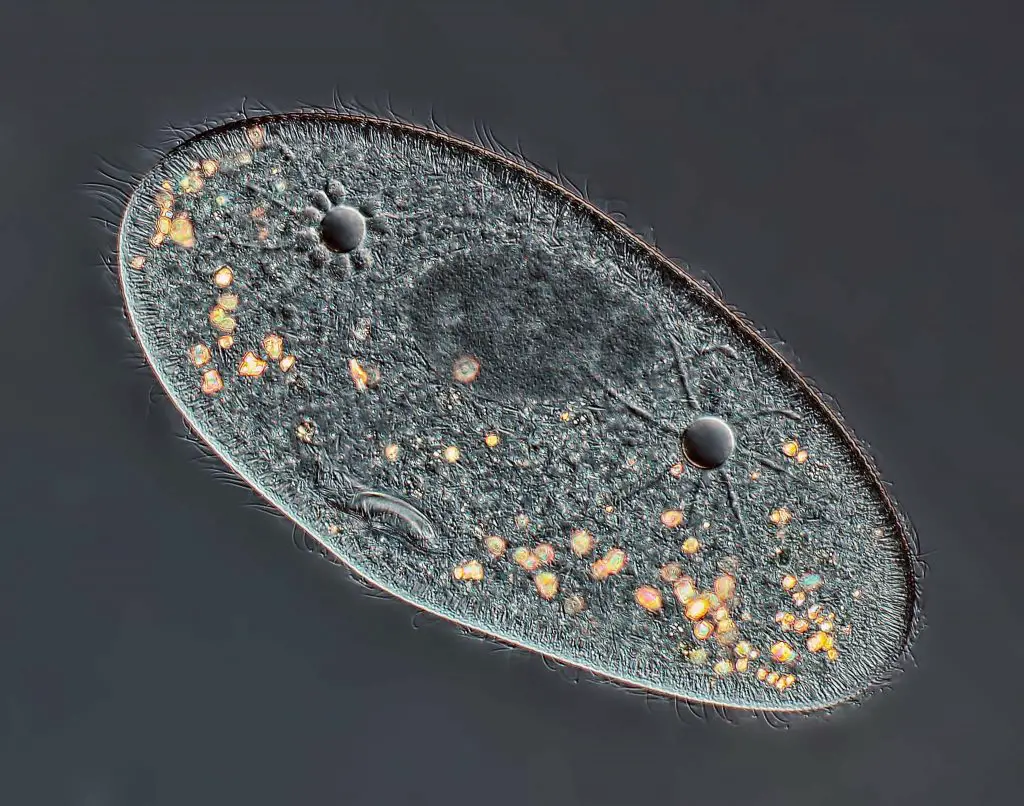
[In this figure] High-definition image of Paramecium showing two star-shaped contractile vacuoles and a macronucleus. This image was taken by Rogelio Moreno from Panama City, Panama, using differential interference contrast (DIC) at 40X magnification. This image received 4th Place in 2013 Nikon Photomicrography Competition.
Food vacuoles
When a paramecium cell collects food through the oral groove and passes through cytostome toward the bottom of cytopharynx, these food materials are encapsulated into food vacuoles. Food vacuoles then fuse with organelles called lysosomes, whose enzymes break apart food molecules and conduct the digestion.
Food vacuoles are non-contractile and are roughly spherical in shape. They serve as an isolated compartment to allow the enzymes to break down only the food particles, but not other organelles. The sizes of food vacuoles vary depending on the amount of food and the progress of digestion. Indigestible debris will be ejected from an opening on the pellicle, called the anal pore or cytoproct.
Related posts
The Biological Classification of Paramecium – Name, History, and Evolution
Pingback: How does paramecium move? – Dr. Biology Questions and Answers
Pingback: What does Paramecium eat? – Dr. Biology Questions and Answers
Pingback: How does Paramecium eat? – Dr. Biology Questions and Answers
Pingback: What does Paramecium use for defense? – Dr. Biology Questions and Answers
Pingback: Is Paramecium unicellular or multicellular? – Dr. Biology Questions and Answers
Pingback: What is the size of the Paramecium? – Dr. Biology Questions and Answers
Pingback: Is Paramecium prokaryotic or eukaryotic? – Dr. Biology Questions and Answers
Pingback: How does Paramecium regulate its water content? – Dr. Biology Questions and Answers