This article covers
Introduction
The genes in your body’s cells play a key role in your health. Indeed, a defective gene or genes can make you sick.
Gene therapy is a very exciting technique with enormous potential to cure many human diseases by fixing problems in our DNA. However, contrary to what the word “therapy” suggests (or what is shown in the illustration below), our DNA is too small to be fixed simply by holding tweezers or scalpels. In reality, we often rely on viruses to deliver DNA molecules into cells for us.
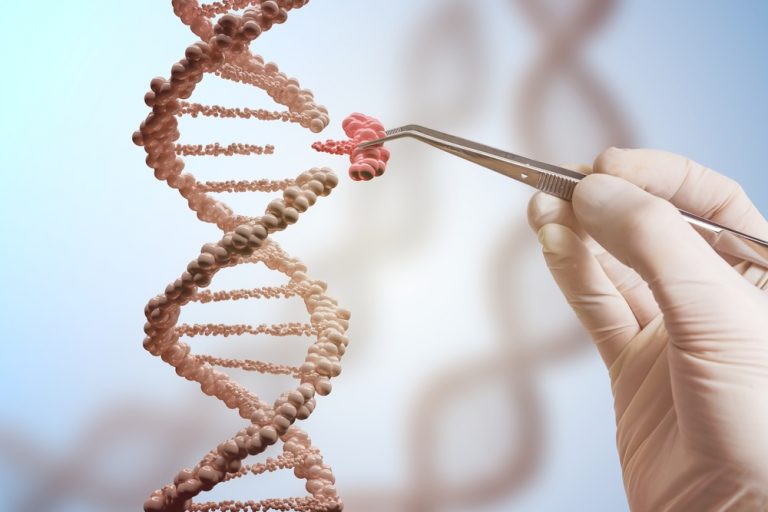
[In this image] A make-believe artwork showcasing gene therapy. It’s purely imaginary, so don’t go trying to replicate it in real life!
Image source: European pharmaceutical review
Are you ready to dive into the fascinating world of gene therapy and learn how scientists are using it to tackle diseases? In this article, we’ll explore the following:
- How gene therapy can fix DNA mutations that cause diseases
- How scientists harness the power of viruses to help us deliver a therapeutic gene
- The exciting new frontier of gene editing and how it works
- Real-life success stories of gene therapy and its promising future
Buckle up and get ready to discover the amazing potential of gene therapy!
What is gene therapy? A quick overview
Gene therapy is a biomedical technique that can help treat genetic disorders, which are diseases caused by problems in our DNA. These diseases happen when a gene mutation permanently changes the DNA sequence, resulting in missing or faulty proteins. By restoring the proper function of these proteins, gene therapy can help alleviate the effects of the mutations.
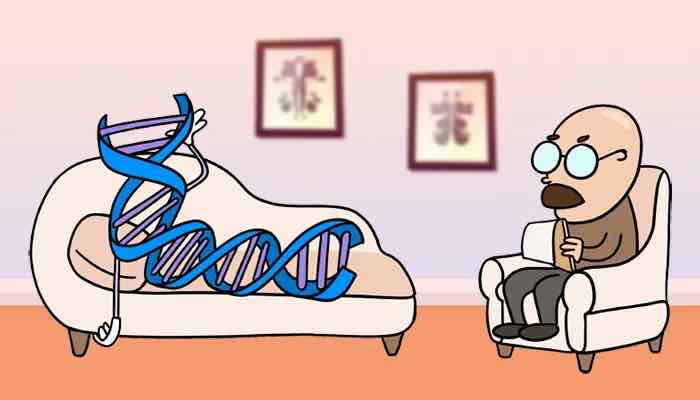
Image source: science me
Gene therapy is a rapidly evolving field with the potential to treat a variety of genetic diseases, such as cystic fibrosis, hemophilia, and some types of cancer.
There are several different approaches to gene therapy:
- Replacing a disease-causing gene with a healthy copy of the gene
- Turning off a disease-causing gene that is not functioning properly
- Introducing a new or modified gene into the body to help treat a disease
What is a gene? – The source code of life
Our genes are encrypted books carrying the secrets of life. They dictate everything from the color of our eyes to our immune system to our likelihood of developing diseases. Our cells read the genes like a manual — provide instructions for assembling proteins. Proteins become the building blocks of the human body, such as the muscles, organs, and immune system.
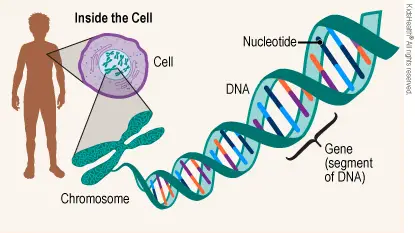
[In this image] Genes and cells are intimately related.
Within the cells of our bodies, there are thousands of genes that provide the information to produce specific proteins that make up the cells.
Image source: TeensHealth
The language of these gene books is written in the nucleotide base pairs (A, T, G, C). The papers of these books are made of DNA molecules.
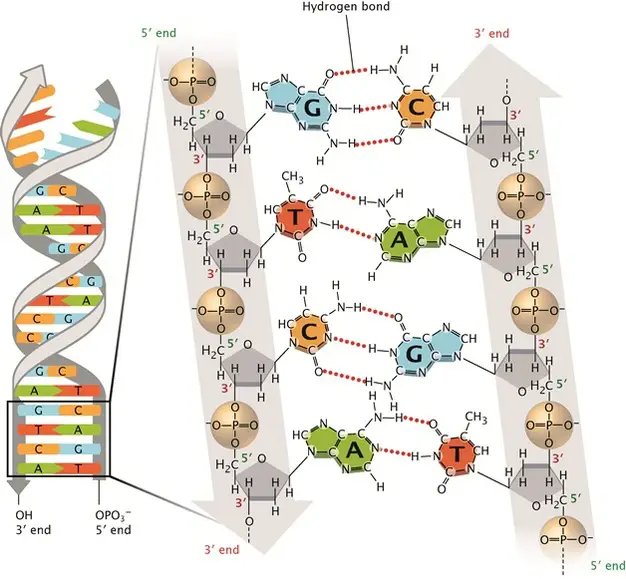
[In this image] DNA double-stranded helix.
The DNA alphabets contain only four letters, which are four nitrogenous base options: Adenine (A), Thymine (T), Cytosine (C), and Guanine (G). Each base can only pair with one other, A with T and C with G. This is called the DNA complementary base pairing rule. By this rule, two complementary DNA strands pair together and form a double-stranded DNA helix.
All of our genes are stored in the cell nuclei, located on 46 pieces of long DNA threads. The entire set of an organism’s genetic materials is called the “genome”. The science of studying genomes is called “genomics”.
Check the article below to learn more about DNA, genes, and chromosomes
When genes go wrong – mutations and genetic diseases
The trouble is genes aren’t always built correctly. When this happens, it changes the gene’s DNA sequences. This is called a mutation. Mutations can be passed down from someone’s parents or can occur for the first time in that person. A mutation in the gene can alter how proteins work. Sometimes, mutations can cause disease. That’s where gene therapy may help.
What happens if the DNA sequences are messed up – Mutations
Mutations (changes in DNA sequences) may derail the genetic information and cause cells to make the wrong proteins. If a mutation occurs in germ cells (or reproductive cells, like sperms and eggs), it will be passed on to the offspring, resulting in an inherited genetic disease. Mutations can also be acquired later in life in somatic cells, which can lead to cancer.
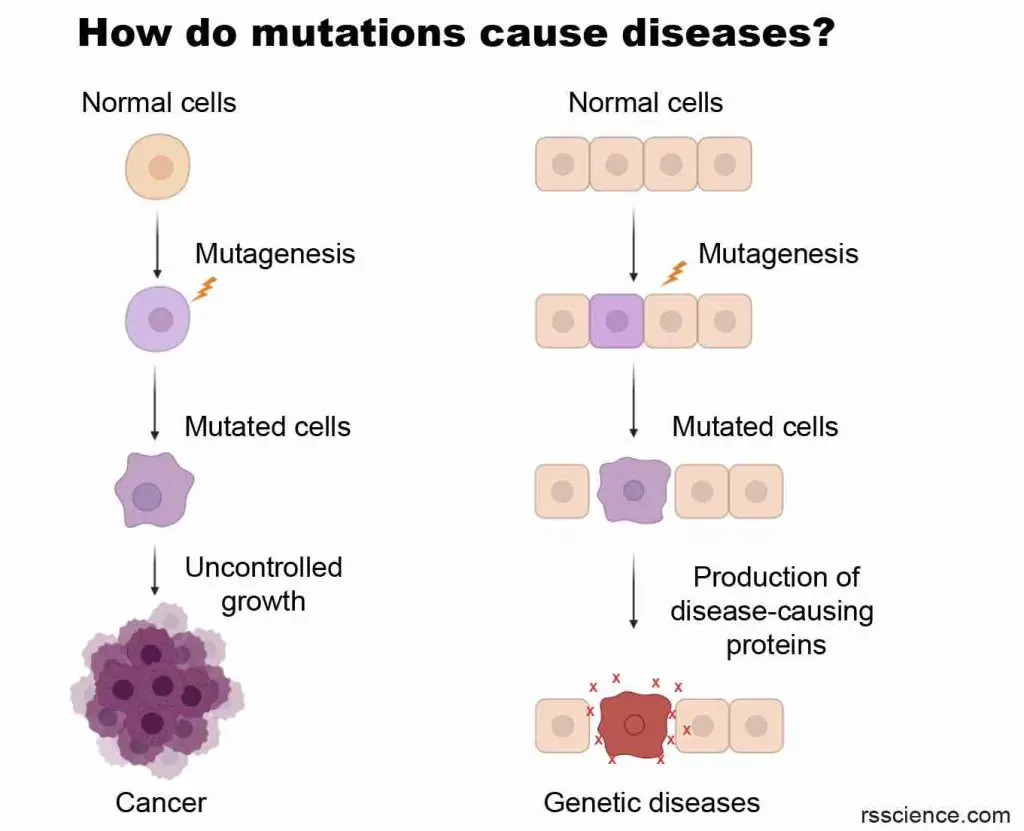
[In this image] Mutations are the major cause of cancers and genetic disorders.
Mutations come in different types, including small-scale DNA changes such as point mutations (affecting a single nucleotide) and frameshift mutations (altering the reading frame). Despite their seemingly minor nature, even the modification of a single base pair can have significant consequences and, therefore, should not be underestimated.
Mutations can also occur on a larger scale. For instance, a large segment of DNA, which may include several genes, could be deleted, duplicated, translocated, or inverted. In some cases, entire chromosomes may be missing or duplicated, resulting in changes in the structure or number of chromosomes that can cause devastating genetic diseases.
Genetic diseases caused by DNA mutations
There are many genetic diseases due to DNA mutations. Most of them are rare diseases. See the table below for examples.
Table. Genetic diseases caused by DNA mutations
Disease Name | Incidence rate | Mutated gene | Description |
Cystic fibrosis | 1 in 2,500 to 3,500 white newborns | Cystic Fibrosis Transmembrane Regulator (CFTR) | Improper salt balance in the lung cells, resulting producing in thick, sticky mucus |
Sickle cell anemia | 1 out of every 365 Black or African-American births | Hemoglobin | Red blood cells become hard and sticky and look like a C-shaped |
Huntington’s disease | 1 in 10,000 people | Huntingtin gene | Neurological illness causing involuntary movements, severe emotional disturbance, and cognitive decline |
Duchenne muscular dystrophy | 1 in 3,500 male births | Dystrophin gene | Progressive muscle degeneration and weakness |
Hemophilia A | 1 in 5,500 male births | Blood clotting factor VIII | Unexplained and excessive bleeding from cuts or injuries |
Hemophilia B | 1 in 25,000 male births | Blood clotting factor IX | Prolonged bleeding from injuries |
Fragile X syndrome | 1 in 10,000 people | Fragile X Messenger Ribonucleoprotein 1 (FMR1) | Developmental delays and learning disabilities |
Severe combined immunodeficiency (SCID) | 1 in 58,000 live births | Several genes of infection-fighting immune cells | Loss of immunity can result in a susceptibility to life-threatening infections |
Down syndrome | 1 in 700 live births | An extra copy of chromosome 21 | Intellectual disability and developmental delays |
More genetic diseases include Tay-Sachs disease, Turner syndrome, Klinefelter syndrome, Angelman syndrome, Prader-Willi syndrome, and Marfan syndrome.
Mutations can cause cancer
Mutations can cause cancer in various ways. Some mutations can activate “oncogenes”, which promote uncontrolled cell growth. Others can inactivate “tumor suppressor genes”, which normally prevent the development of cancer. Additionally, mutations can affect DNA repair mechanisms or cause chromosomal abnormalities that can lead to further mutations and genomic instability. These accumulated mutations can result in the uncontrolled proliferation of cells and the development of cancerous tumors.
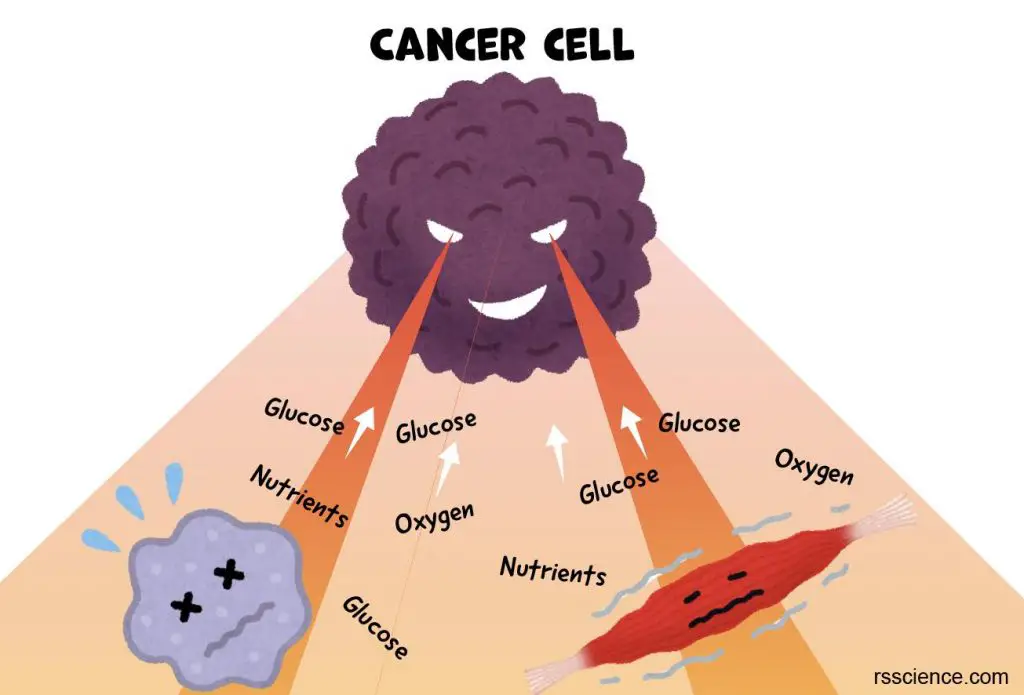
[In this image] Cancer cells plunder the nutrients from surrounding cells and tissues to sustain the rapid growth of tumors.
Extended reading: What does the Cori cycle do?
Mutations can be acquired in our somatic cells over the course of our lives. Exposure to toxins and smoking can increase the risk of cancer by introducing carcinogens into the body. Carcinogens are substances that have the potential to harm DNA, making it more probable for mutations to arise.
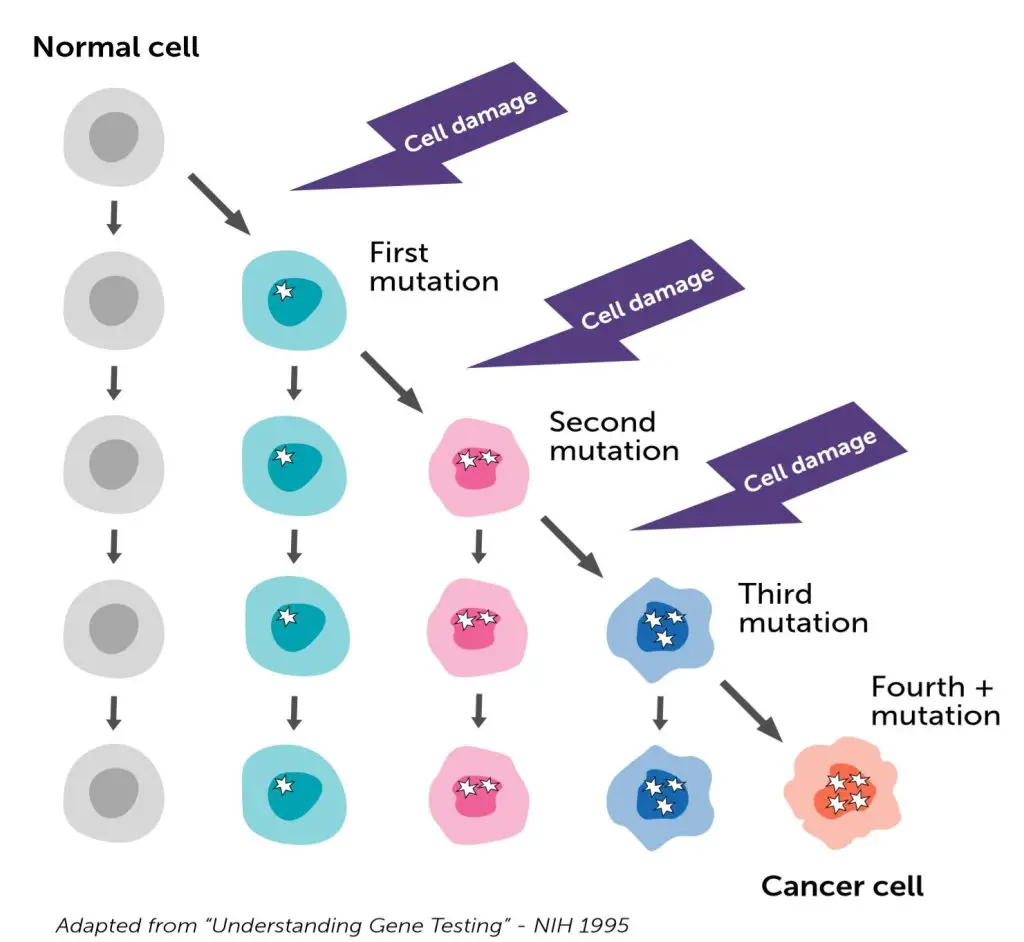
[In this image] Cancer is caused by gene mutations that build up over time, leading cells to grow uncontrollably and become cancerous. It can take years for enough mutations to accumulate for cancer to develop.
Image source: Facing Hereditary Cancer Empowered
Certain types of cancer are inherited, which means the genetic mutations that cause cancer can be passed down from parents to their children. These mutations are present in all cells of the body and increase the chance of developing cancer over time. People with a family history of certain cancers are at a higher risk of developing them.
Examples of inherited cancers include neurofibromatosis, BRCA1 and BRCA2 mutations (linked to breast and ovarian cancer), Lynch syndrome (linked to colorectal and other types of cancer), Von Hippel-Lindau syndrome (linked to tumors and cysts), and Retinoblastoma (eye cancer).
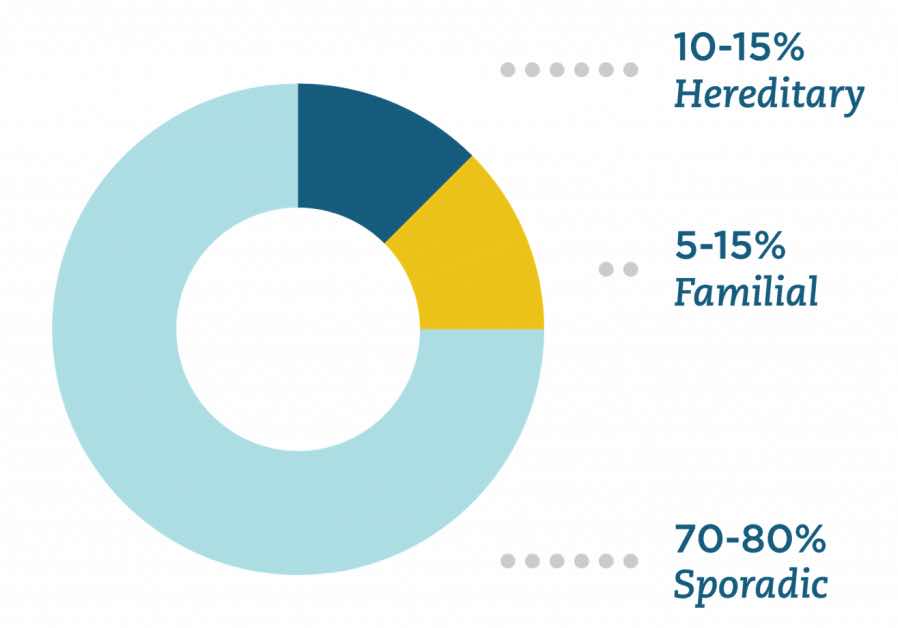
[In this image] Hereditary cancer is caused by a gene passed down from parents. Familial cancer happens more often in families than is expected from chance alone, but we don’t know why yet. Sporadic cancer happens when a person’s cells change by themselves over time.
Image source: Color
Your DNA may also determine your susceptibility to non-genetic diseases
While our DNA determines many of our inherited traits and characteristics, it can also increase our susceptibility to certain diseases, even if genetics is not the sole cause.
As we unlock the secrets of the human genome (the complete set of human genes), we are learning that nearly all diseases have a genetic component. Many diseases, such as heart disease, diabetes, cancer, and neurodegenerative diseases, are caused by a combination of genetic and environmental factors. Our genes can affect how our bodies handle substances like cholesterol and glucose, and can also impact our immune system, making us more or less likely to get certain illnesses. However, our lifestyle choices, such as diet, exercise, and smoking, can also affect our chances of getting these diseases.
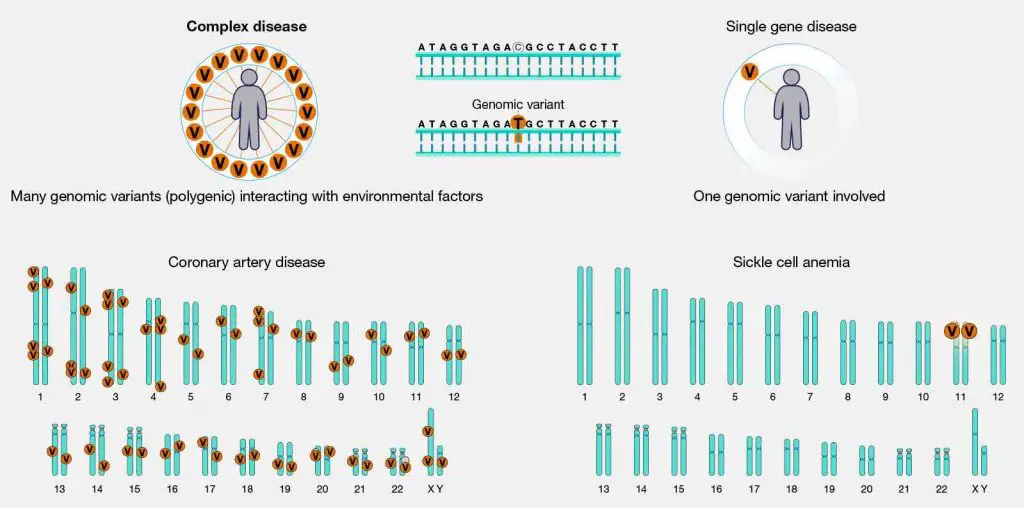
[In this image] Complex diseases, also known as multifactorial diseases, result from the interaction of multiple genes and environmental factors. For example, the genes associated with coronary artery disease are found on all chromosomes, with each gene contributing only a small chance of developing the disease. These genes interact with each other in complicated ways. In contrast, sickle cell anemia is a simple genetic disease caused by a mutation in a single gene. Examples of complex genetic diseases include heart disease, diabetes, aging, and cancer.
Image source: NIH
Scientists are still trying to understand how our genetics are involved in these diseases. It’s possible that several genes work together to increase the risk of developing a disease, with each gene contributing only a small amount. One type of genetic difference between individuals is called Single Nucleotide Polymorphisms (SNPs), which are usually harmless, but some may contribute to a person’s risk of developing certain diseases. In the future, personalized medicine may use information from your unique genetic makeup to design a therapy that is best suited for you.
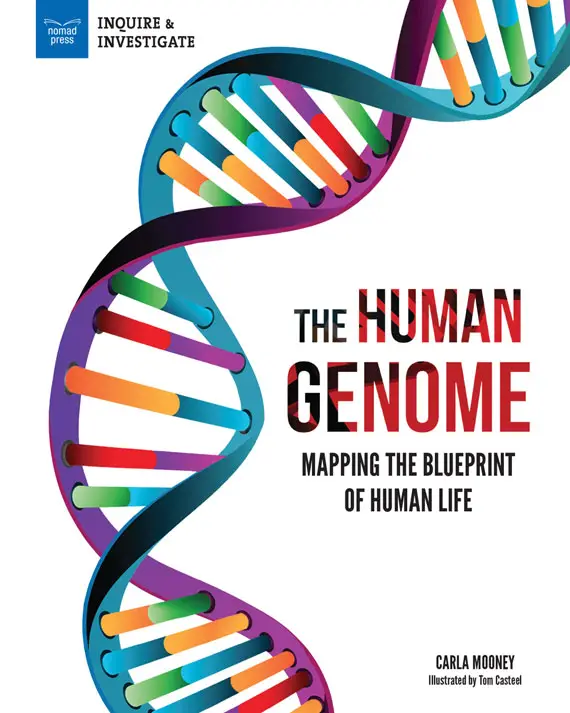
[In this image] The completion of the Human Genome Project provides a map to help us understand the genetic makeup of our bodies. This can help us create personalized therapies tailored to an individual’s unique genetic profile.
Image source: nomadpress
Fix a broken gene – How does gene therapy work?
There are many kinds of genetic diseases, and each has its own unique cause of DNA mutations. When trying to fix these broken genes using gene therapy, we have to choose the right way to do it. Below are different methods that scientists commonly use.
Gene addition
When a disease is caused by a single gene, such as hemophilia, the most direct way to treat it is through a method called gene addition. This involves adding a healthy copy of the gene to the patient’s cells.
To carry out gene addition therapy, a specially engineered virus or another type of vector is needed to deliver the new gene into the cells. The new gene produces a protein that the body was unable to produce before, which helps the body to function properly. This therapy is typically a one-time treatment that can provide long-lasting effects.
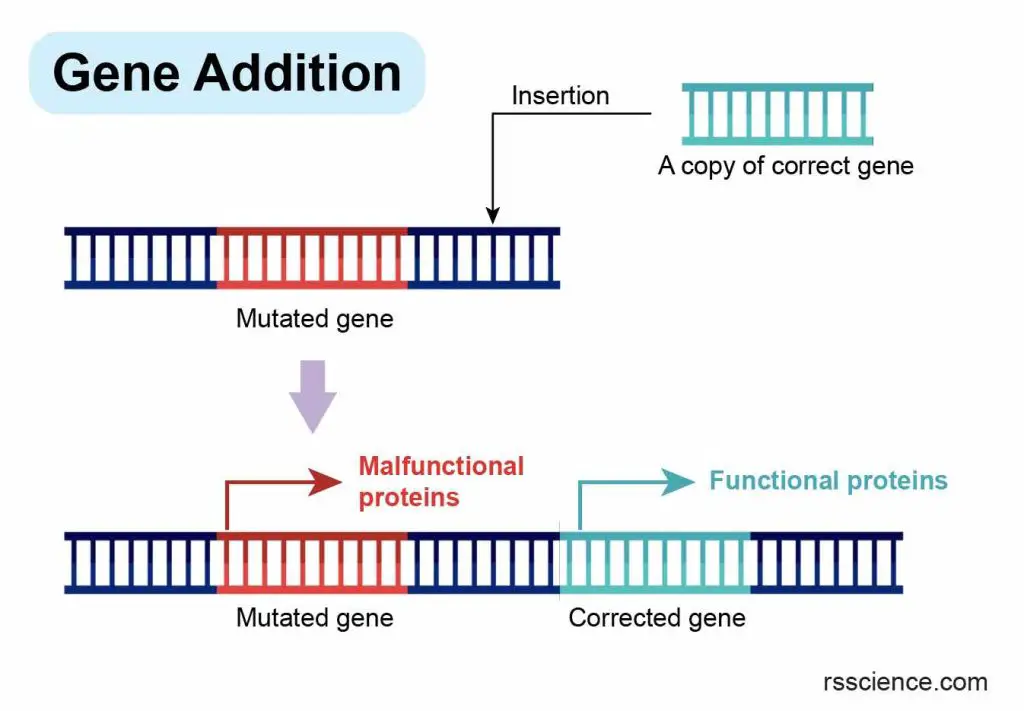
[In this image] The concept of gene addition.
One copy (or multiple copies) of a healthy gene is introduced to the cells carrying a faulty gene. Typically, a viral vector is used to deliver the corrected gene sequence to the cells. Once inside the cell, the corrected gene is inserted into the genome and begins producing functional proteins, thereby compensating for the dysfunction caused by the mutated gene. This approach leads to therapeutic benefits in treating genetic diseases.
Gene silencing
Gene silencing, also known as RNA interference (RNAi) therapy, is a type of gene therapy that “turns off” a specific gene in the body. This is done by introducing small RNA molecules that bind to and destroy the messenger RNA molecules that produce the disease-causing proteins. This therapy has the potential to treat a range of diseases, including sickle cell disease, viral infections, and some cancers. However, scientists are still working to develop safe and effective methods to deliver the therapy and choose the right genes to target.
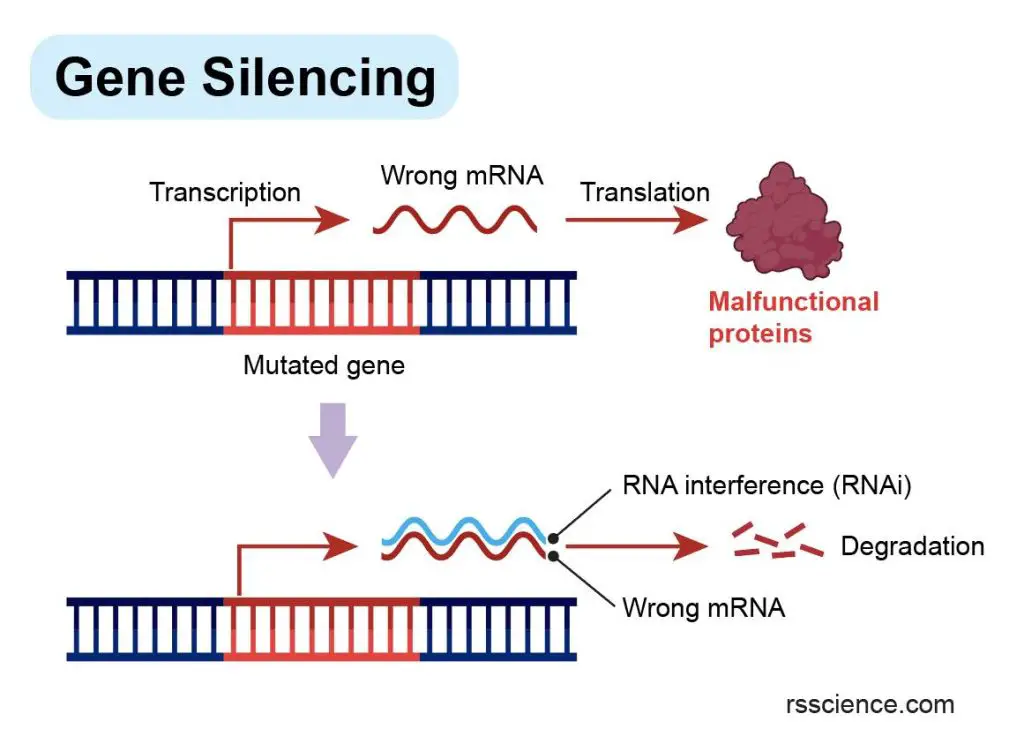
[In this image] The concept of gene silencing.
Gene silencing is a potential therapeutic approach for genetic diseases caused by abnormal proteins resulting from mutated genes. In such cases, the most effective treatment is to switch off the faulty gene. This can be achieved by introducing RNA interference (RNAi) into the cells, which targets and degrades the incorrect mRNA responsible for producing the harmful proteins.
Gene editing
Gene editing can be used for gene therapy by precisely adding, removing, or replacing specific sections of DNA that are causing disease. This is done by making cuts to the DNA at the exact spot where it is not functioning correctly. To do so, scientists can use different types of tools, including CRISPR-Cas9, zinc finger nucleases (ZFNs), and transcription activator-like effector nucleases (TALENs), to make precise cuts in the DNA. Once the DNA is cut, the cell’s natural repair mechanisms take over to fix the DNA. Gene editing can disrupt unwanted DNA elements or insert new sequences to fix faulty genes, resulting in permanent genetic changes.
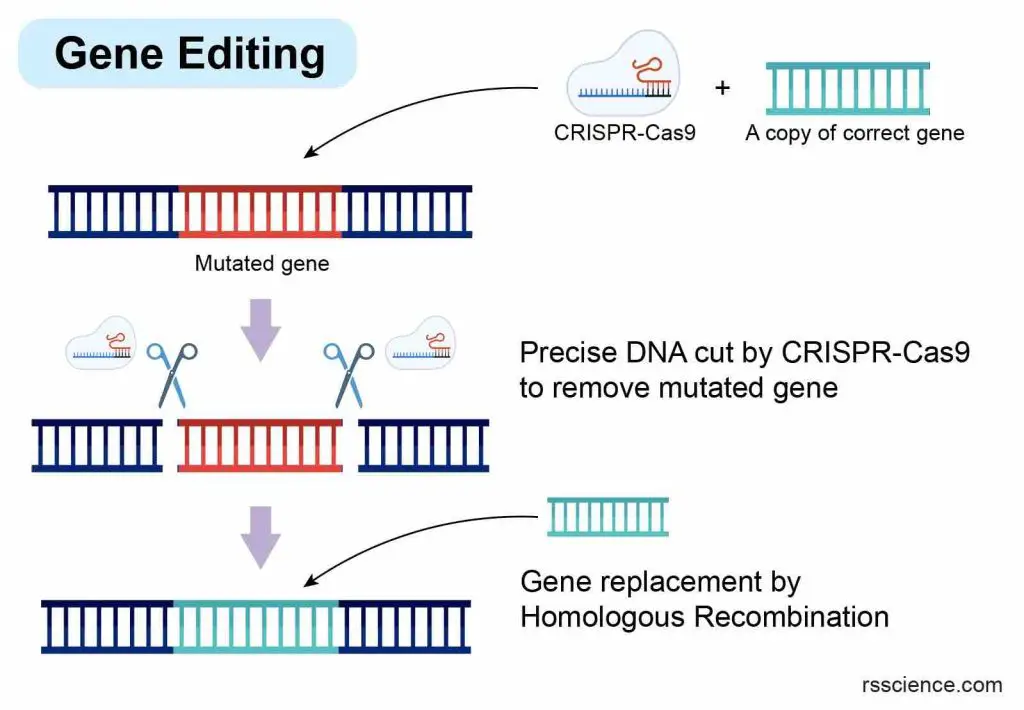
[In this image] The concept of gene editing with CRISPR-Cas9 to modify genes.
Cells carrying a faulty version of the gene are targeted with CRISPR-Cas9, which acts as molecular scissors to precisely cut out the mutated gene. At the same time, a corrected copy of the gene sequence is inserted into the cut sites to replace the mutated version.
Gene editing can potentially treat a wide range of genetic disorders, including cystic fibrosis, sickle cell anemia, and Huntington’s disease. However, there are still significant technical and ethical challenges associated with gene editing, including concerns about off-target effects (making unwanted changes to other parts of our DNA) and unintended consequences. Ongoing research is focused on developing safe and effective methods for using gene editing to treat genetic diseases.
Base editing
Base editing is a new tool modified from CRISPR-Cas9 gene editing. Base editing can make precise changes to individual DNA base pairs (i.e., A-to-G or T-to-C).
Base editing is particularly useful for correcting genetic diseases caused by point mutations. It can target single-point mutations and correct errors in genetic code. These changes can also silence a disease-causing gene or help activate a specific gene. Base editing has the potential to treat a wide range of genetic disorders, including sickle cell anemia, cystic fibrosis, and some types of cancer.
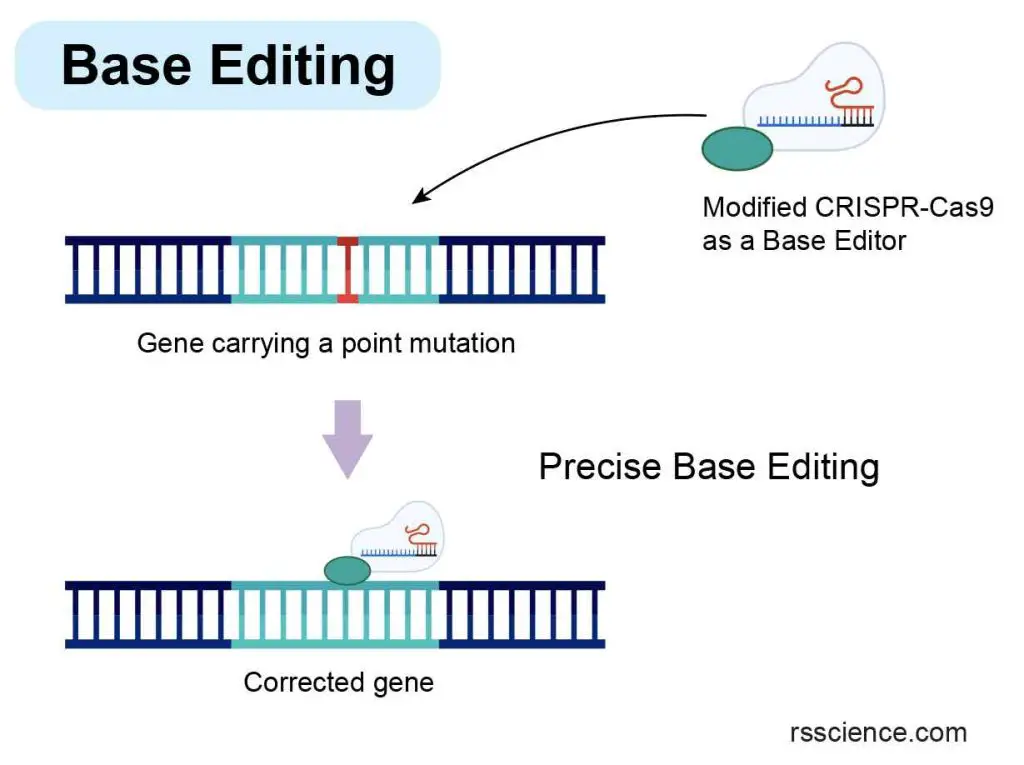
[In this image] The concept of Base Editing, a technique that enables the rapid correction of point mutations.
To achieve this, a modified CRISPR-Cas9 system is introduced into cells as a base editor. Unlike traditional CRISPR-Cas9, base editing does not require the cutting of the DNA molecule. Instead, it utilizes a specialized enzyme to directly convert a single DNA base (nucleotide) into another without altering the overall structure of the DNA molecule.
How to deliver gene therapy?
Gene therapy requires efficient delivery of the correct genetic materials to specific cells in certain organs, such as the brain or lungs, to produce the desired therapeutic effects.
The challenge is that we can’t just use a syringe to inject DNA into each cell one by one. Therefore, how can we deliver gene therapy?
Scientists have come up with cool ways to do so using their knowledge of biology, chemistry, and physics. Let’s see how these tools can efficiently deliver genetic materials into a bunch of cells at once, so we can get these therapeutic benefits we’re after. I guarantee that’ll blow your mind!
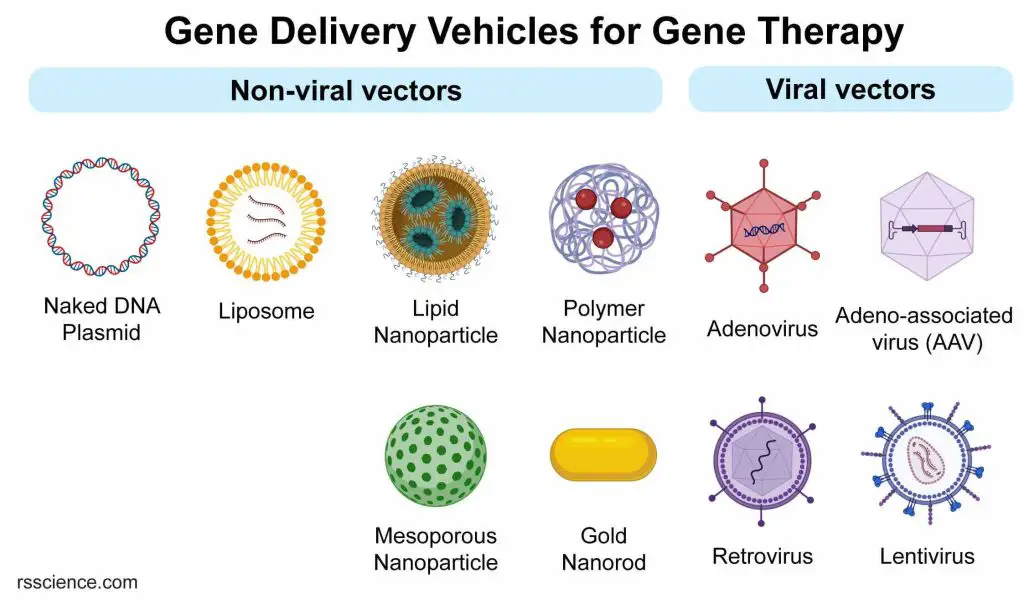
[In this image] Scientists use a tool called a “vector” to put new genes into cells. These vehicles can be classified into non-viral or viral vectors. The most commonly used vectors are viruses because they are good at delivering genes into cells.
Naked DNA
Naked DNA delivery for gene therapy involves directly injecting the therapeutic DNA into the patient’s body without any carriers. The goal is to get the naked DNA into the target cells so it can express and produce the desired therapeutic protein. While this method is simple, it’s not very effective since naked DNA can be easily broken down by the immune system. As a result, scientists have moved on to other methods that we will talk about later.
Electroporation is a method to get naked DNA into cells in a petri dish. This method involves giving the cells a brief but powerful electric shock that creates tiny holes in their membranes, allowing the DNA to enter. Electroporation is commonly used in cell engineering to deliver genetic materials.
Usually, circular DNA molecules (plasmid DNA) that can be massively produced in bacteria are used to carry therapeutic genes into human cells. In some cases, linear DNA or mRNA can also be used to deliver therapeutic genes.
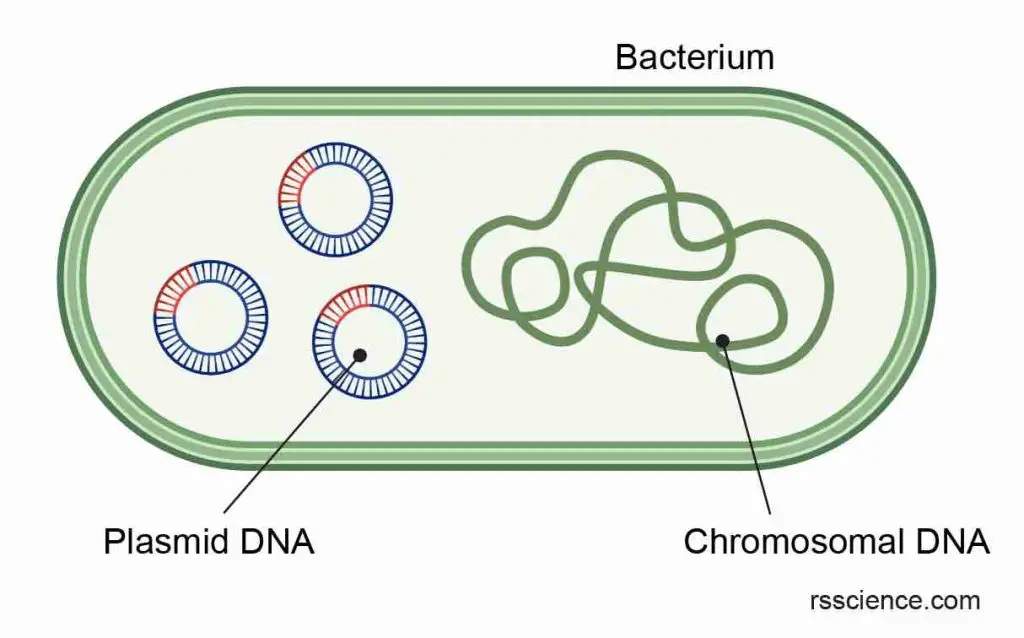
[In this image] Plasmid DNA is a small, circular piece of genetic material found in some bacteria. It is separate from the bacterial chromosomal DNA, and can replicate independently within the cell. Plasmids can carry genes that provide benefits to the host bacterium, such as antibiotic resistance. Through recombinant DNA technology, scientists can manipulate plasmids and leverage them as vectors to introduce novel genetic material into cells, aiding in the development of gene therapies and vaccines.
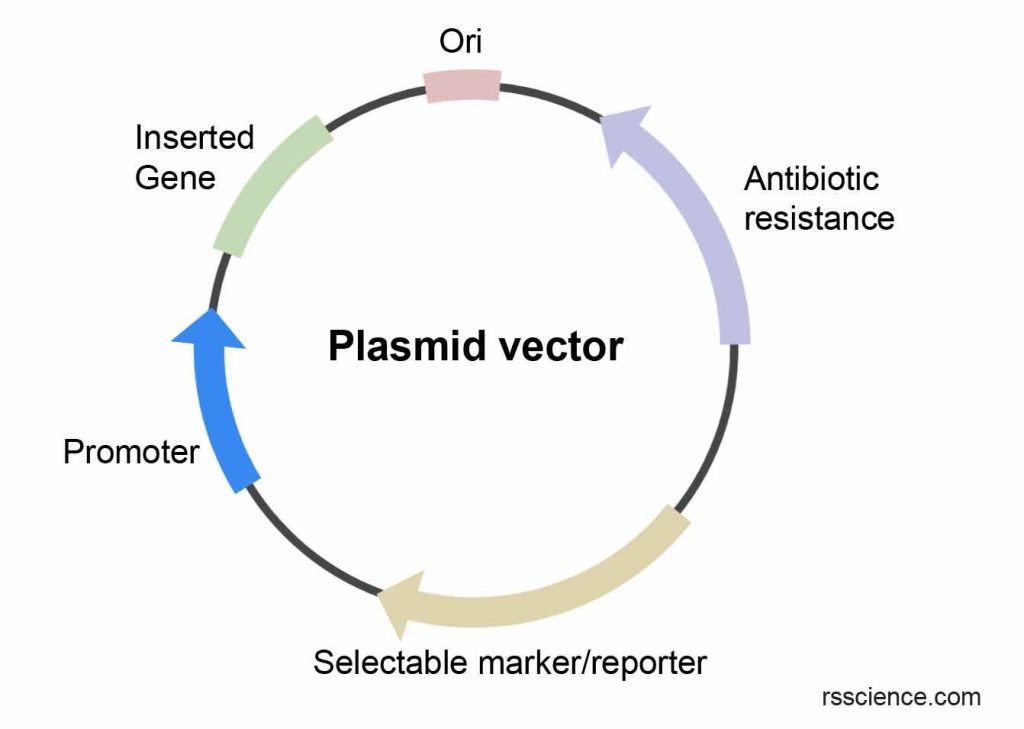
[In this image] A plasmid vector commonly consists of several components.
The Origin of replication (Ori) is a DNA sequence that enables bacteria to replicate more plasmids. Antibiotic resistance is used to select bacteria carrying the plasmid. The Inserted gene is the gene of interest delivered for gene therapy. The Promoter functions as the driver to express the inserted gene. Finally, a Selectable marker or reporter is utilized to monitor or select target cells following successful gene delivery.
Liposomes
Naked DNA is not an effective method for gene therapy due to the cell membrane’s barrier properties, which consist of two lipid layers that hinder the passage of large molecules such as DNA.
An alternative approach is to use liposomes, which are tiny, spherical carriers made up of the same molecules as the cell membrane. By encapsulating therapeutic DNA within the liposomes (like a capsule), membrane fusion can occur, releasing the DNA directly into the cells.
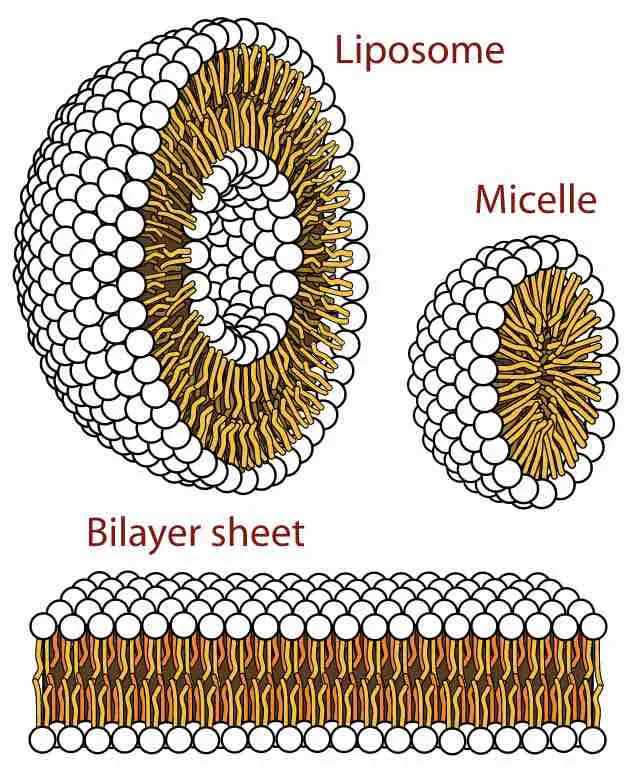
[In this figure] The three main structures phospholipids form in solution: the liposome (a closed bilayer), the micelle, and the bilayer.
Image source: wiki
Extended Reading: Cell membrane – definition, structure, function, and biology
Liposomes are introduced into the body through injection or inhalation, depending on the target cells and organs. Once inside, liposomes can also protect the DNA from degradation by the immune system and help it enter cells more efficiently.
Once inside, the liposomes fuse with the cell membrane, releasing the therapeutic DNA into the cells where it can produce the desired therapeutic protein. Liposomes are biocompatible and less likely to cause an immune response. But liposomes still have some drawbacks, like poor stability and difficulty in targeting specific cells or organs. Researchers are working to improve liposome-based gene therapy delivery systems.
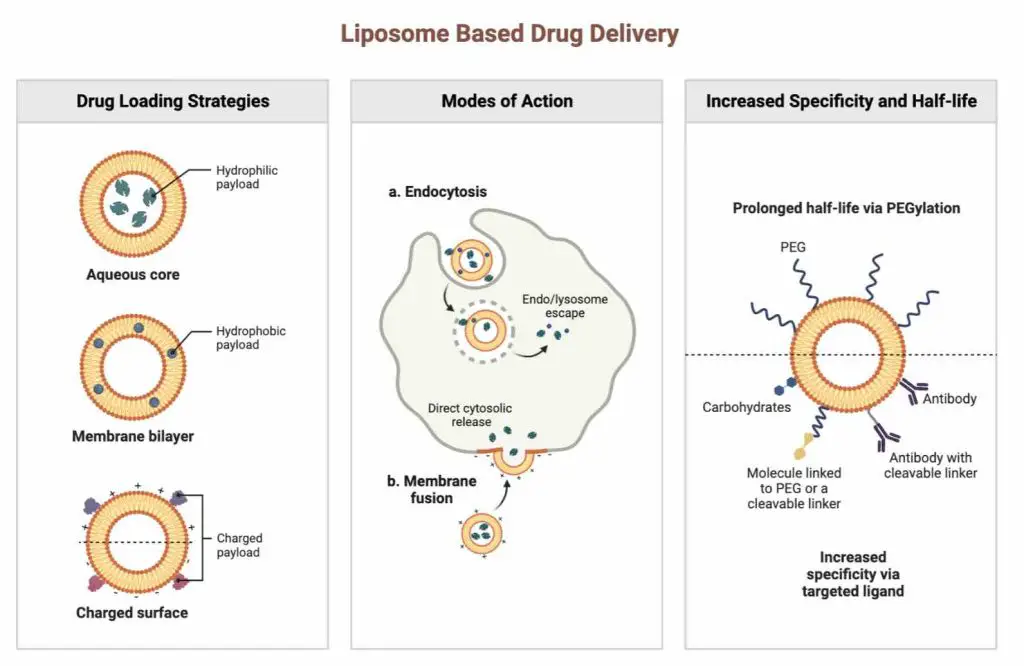
[In this image] The concept of using liposomes for drugs or DNA delivery.
Image credit: Biorender
Nanoparticles
Nanoparticles are tiny particles (measured in nanometers) that can be engineered to carry and deliver therapeutic DNA into cells. These particles can be made of different materials, such as metals, polymers, lipids, and proteins.
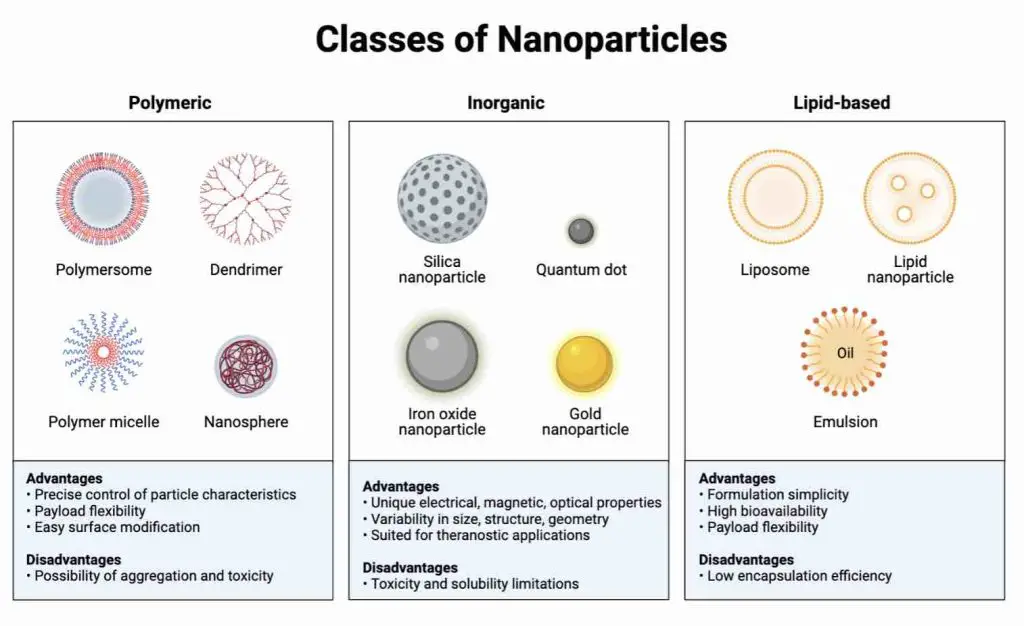
[In this image] Common classifications of nanoparticles.
Image credit: Biorender
Nanoparticles for gene therapy are injected into the bloodstream or target tissue. Once in the body, the nanoparticles can be taken up by cells and release the therapeutic genes.
Nanoparticles offer a significant benefit by enabling targeted delivery to specific cells or tissues. By modifying the surface properties of nanoparticles, researchers can engineer them to bind to particular cell types selectively.
Additionally, scientists can customize nanoparticles to release their contents only when specific stimuli are detected, such as pH level, heat, light, or magnetic field, to control the delivery timing. This advanced approach enhances the precision and effectiveness of gene delivery.
Note: Liposomes and nanoparticles are together referred to as “non-viral” vectors —or delivery vehicles.
Viral vectors
When it comes to delivering foreign DNA into cells, viruses are undoubtedly experts. Viruses have evolved to be incredibly effective at hijacking cells by inserting their viral DNA or RNA into hosts, which helps them multiply and spread. Unfortunately, we often experience this firsthand through illnesses like COVID-19 and the flu.
Clever scientists are capitalizing on viruses’ ninja-like abilities and modifying them into delivery vehicles for gene therapy. These genetically modified viruses have been altered to remove their harmful properties and to make them suitable for therapeutic purposes.
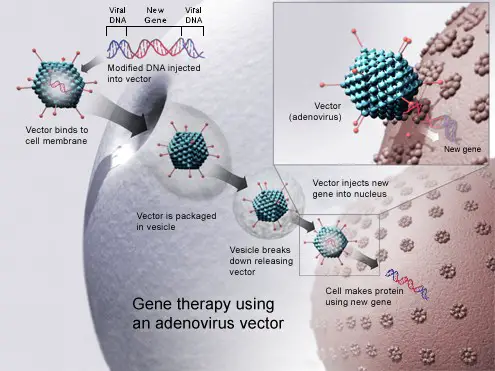
[In this image] Viral vectors are typically used for gene therapy due to their ability to integrate DNA into the host’s genome.
Image source: wikimedia
For example, lentiviruses that cause AIDS are engineered for gene therapy. Scientists removed the viral genes necessary for HIV propagation and replaced them with a therapeutic copy of factor IX. These modified viruses retain their exceptional infectivity to human cells, thanks to the properties of virus shells. However, instead of delivering viral genes, once inside the patient’s cells, they transfer the factor IX gene, which has the potential to cure hemophilia B.
Different types of viruses, such as adenoviruses, adeno-associated viruses (AAVs), retroviruses, and lentiviruses, are used as vectors in gene therapy. Each type of viral vector has specific advantages and disadvantages in terms of safety, efficiency, and immune response.
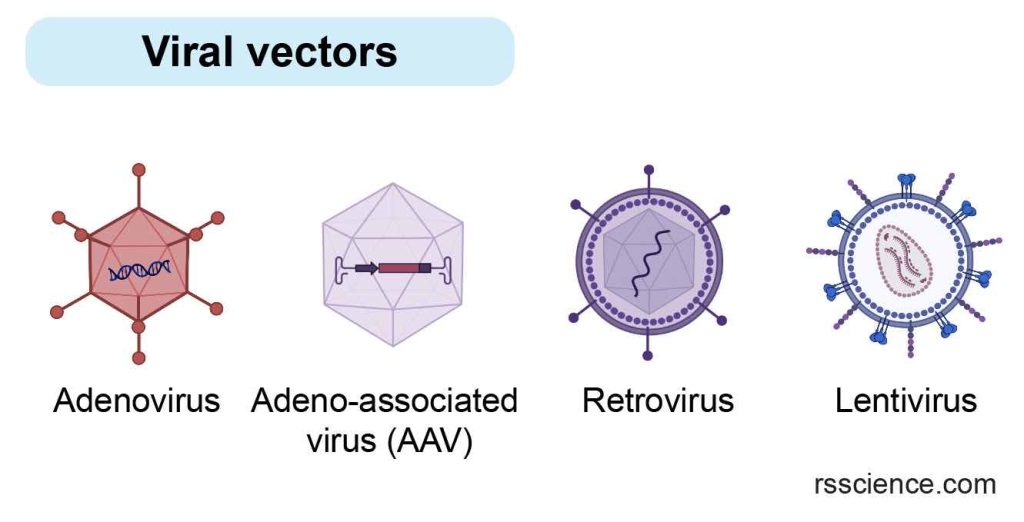
[In this image] Adenoviruses, adeno-associated viruses (AAVs), retroviruses, and lentiviruses are the most common viral vectors for gene therapy.
Table. Comparison between common viral vectors for gene therapy
Virus type | Adenoviruses | Adeno-associated viruses (AAVs) | Retroviruses | Lentiviruses |
Genetical material | dsDNA | ssDNA | ssRNA | ssRNA |
Genome size | 36 kb | 8.5 kb | 7-11 kb | 8 kb |
DNA insert size | >8kb | 4 kb | 7 kb | 8.5 kb |
Infection | Dividing and non-dividing cells | Dividing and non-dividing cells | Dividing cells | Dividing and non-dividing cells |
Host genome interaction | Episomal | Episomal; site-specific integration | Inserted into host genome | Inserted into host genome |
Expression | Transient | Stable | Stable | Stable |
Drawbacks | Elicits strong antiviral immune response | Requires helper virus for replication; difficult to produce pure viral stocks | Insertional mutagenesis potential | Insertional mutagenesis potential |
Popularity | ☆ | ☆☆☆☆☆ | ☆ | ☆☆☆ |
Note: ss = single-stranded; ds = double-stranded
One of the challenges of using viral vectors in gene therapy is the risk of the immune response or toxicity caused by the viral vector itself. Therefore, extensive safety studies and clinical trials are conducted to evaluate the safety and effectiveness of viral vectors before they are approved for use in gene therapy.
Types of gene therapy
ex-vivo vs. in-vivo gene therapy
Gene therapy can be performed in one of two ways — outside the body (ex vivo) or inside the body (in vivo).
What is ex-vivo gene therapy?
Ex vivo gene therapy involves collecting cells from a patient and introducing a vector containing the therapeutic gene into those cells in a laboratory setting. Once the cells have been corrected, they are transplanted back into the patient. This type of gene therapy is suitable for diseases that can be treated by fixing a certain portion of cells (not every cell). Ex vivo gene therapy is considered safer since the vectors are used in a controlled environment outside the patient’s body.
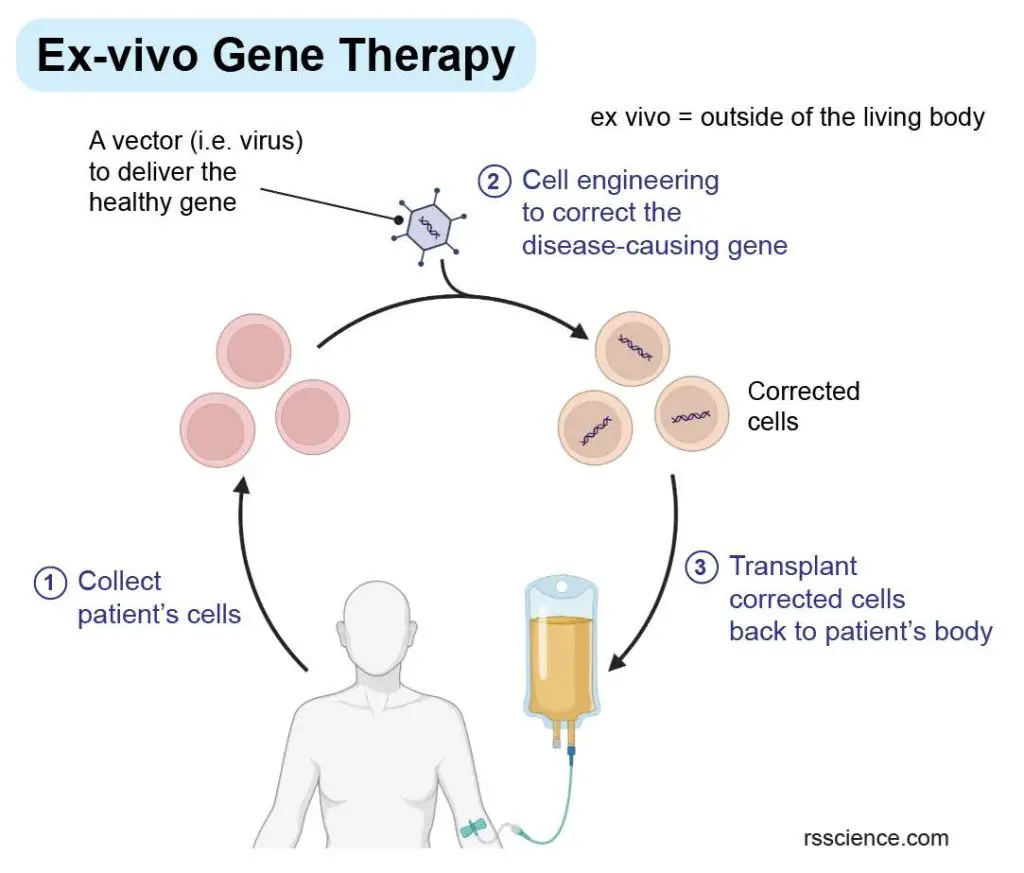
[In this image] The concept of ex-vivo gene therapy.
Ex-vivo gene therapy involves modifying cells outside of the body before reintroducing them to the patient.
One of the first uses of ex vivo gene therapy was in the treatment of a young girl with a rare genetic disease, adenosine deaminase deficiency, or ADA deficiency, which is the most common severe combined immunodeficiency (SCID). People with this disorder are missing the ADA enzyme, which affects the immune system. As a result, individuals with ADA deficiency do not have a healthy immune system to fight off infections.
To treat this, doctors took bone marrow stem cells (cells can differentiate into all kinds of blood cells) from the patient’s body, added a healthy gene to them in a laboratory, and put them back into the patient’s bloodstream. This helped the patient’s immune system work properly, but the treatment needed to be repeated several times.
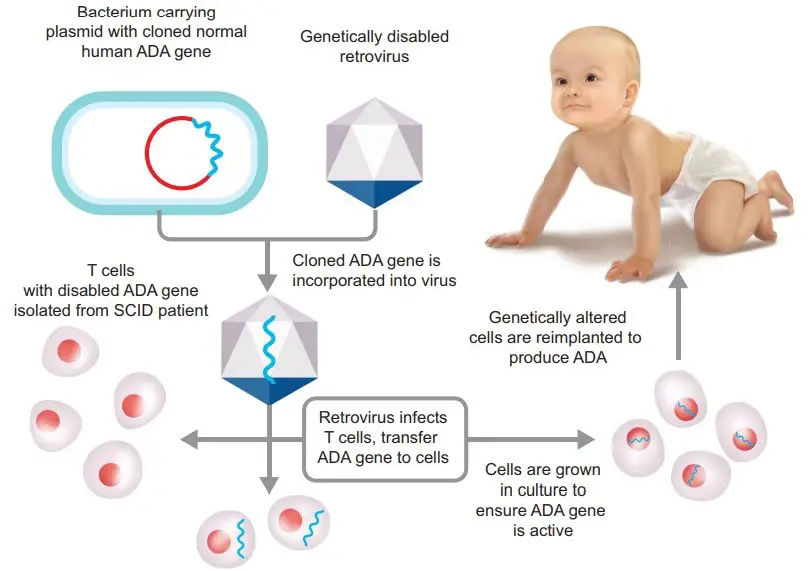
[In this image] The first clinical gene therapy was given in 1990 by French Anderson to a four-year-old girl with adenosine deaminase (ADA) deficiency.
Image source: BrainKart.com
Ex vivo gene therapy is a great option to combine with induced pluripotent stem cell (iPSC) therapy, which involves using a patient’s own cells to treat diseases. Scientists have used this approach in a fascinating study to treat hemophilia A, a blood clotting disorder. Instead of taking blood or tissue biopsies, scientists collected urine samples from hemophilia A patients to culture their epithelial cells.
Using these cells, scientists generated iPSCs from epithelial cells in the laboratory that can grow and differentiate into various cell types depending on the patient’s needs. They introduced a healthy copy of the FVIII gene, which encodes the blood clotting factor FVIII, into the patient’s iPSCs to fix hemophilia A
The corrected iPSCs were differentiated into endothelial cells, which form blood vessels. These cells were then transplanted back into the patient’s body, forming a bioengineered tissue full of blood vessels that could produce FVIII directly into the patient’s bloodstream, thus curing the hemophilia A problem.
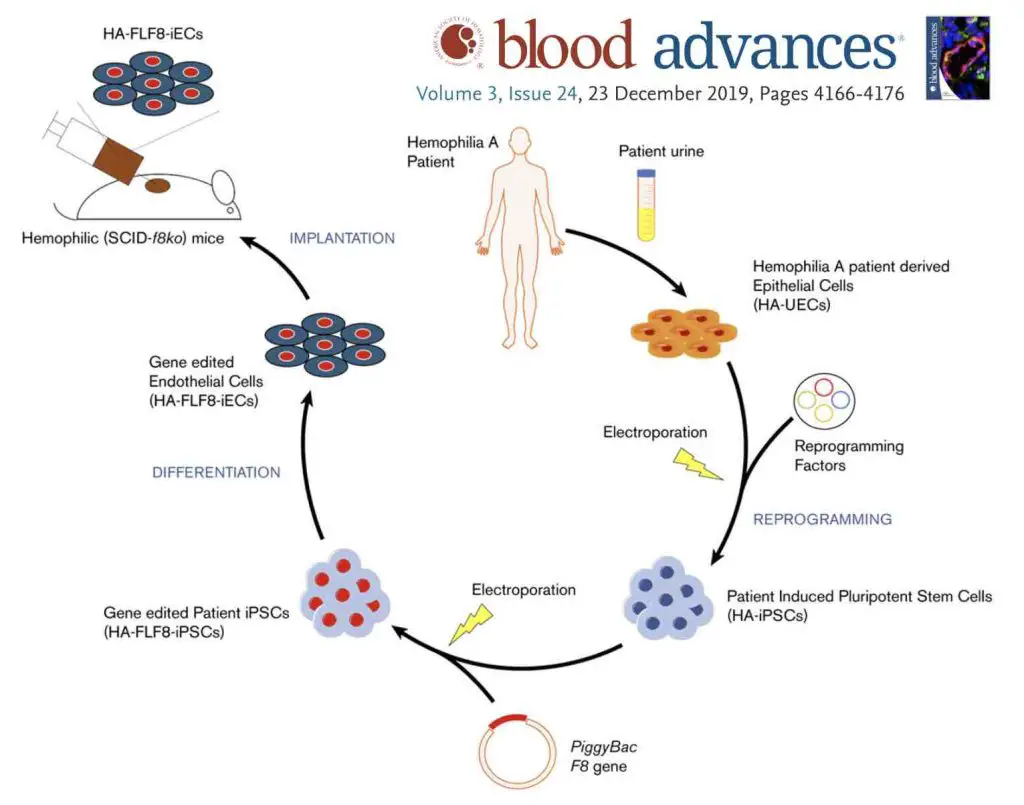
[In this image] A research combining iPSC and ex vivo gene therapy to treat hemophilia A.
Image source: Neumeyer J., et. al., Blood advances, 2019
What is in-vivo gene therapy?
When gene therapy is performed in vivo (inside the body), the therapeutic payload, such as a gene, gene silencer, or gene editor, is delivered directly into the patient’s body using a vector. This vector is often a virus that can efficiently deliver the gene to the organ or tissue affected by the disease.
In-vivo gene therapy is a simple procedure and can be done in an outpatient setting, but it comes with a higher risk. We cannot entirely control where the genetically-modified viruses go, which can pose some unknown risks to the patient’s health.
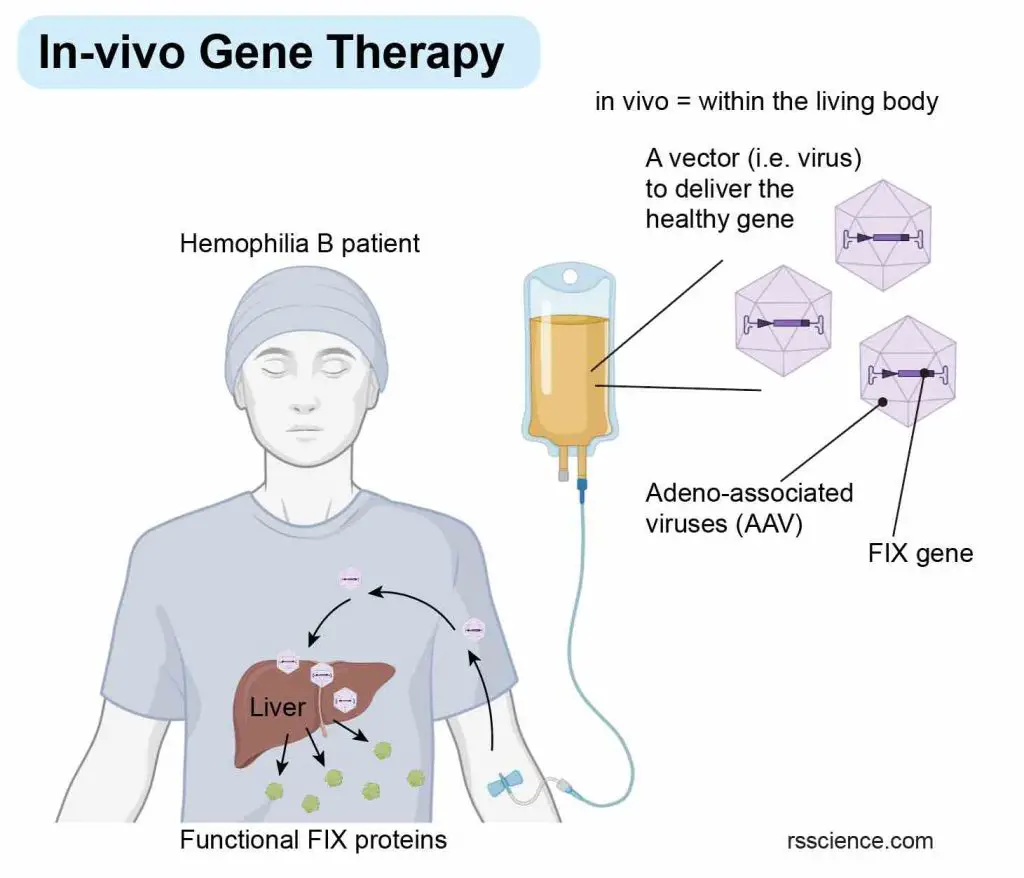
[In this image] The concept of in-vivo gene therapy.
During in vivo gene therapy, done inside the body, the vector with the gene of interest is introduced directly into the patient and taken up by the patient’s cells. In this case, adeno-associated viruses (AAV) carrying a healthy factor IX (FIX) gene are used to restore the blood clotting function in a hemophilia B patient.
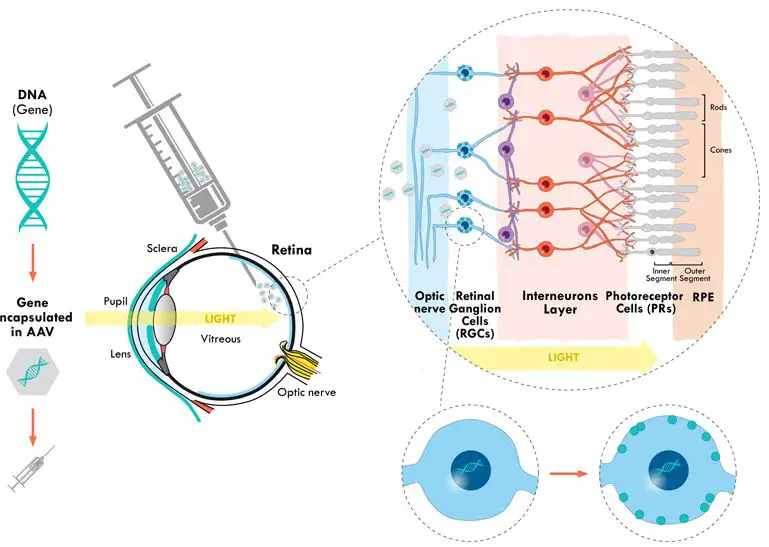
[In this image] Luxturna (by Spark Therapeutics) became the first gene therapy for inherited blindness to receive FDA approval back in 2017. This therapy is designed to treat patients with mutations in a gene called RPE65, which encodes a retinal protein necessary for the eye to respond to light. Viruses carrying a healthy copy of RPE65 are injected directly into patients’ eyes. A single injection in each eye is enough to improve vision for at least three years.
Somatic vs. germline gene therapy
Somatic gene therapy targets only diseased body cells. This is distinct from germline gene therapy which may one day edit sperm and egg cells. Due to the considerable risks and ethical concerns, all gene therapy approved or tested now fall under the domain of somatic gene therapy.
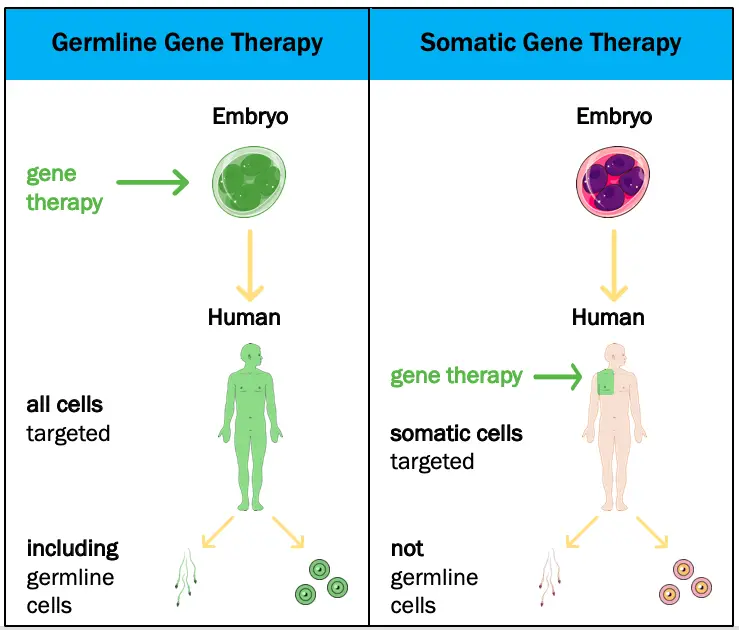
[In this image] Germline vs. Somatic gene therapy.
Image source: TheGIST
Gene therapy for cancer
Ever since scientists realized that changes in DNA cause cancer, gene therapy was proposed for cancer treatment. However, correcting mutated genes in every cancer cell is not feasible. Even if one cell escapes from gene correction, it can proliferate and form a new tumor. For this reason, to fight cancer through gene therapy, scientists must think creatively and look beyond conventional approaches. Here are three highly promising techniques.
Suicide gene therapy
Suicide gene therapy involves introducing a gene into cancer cells that causes them to self-destruct when triggered by a specific drug.
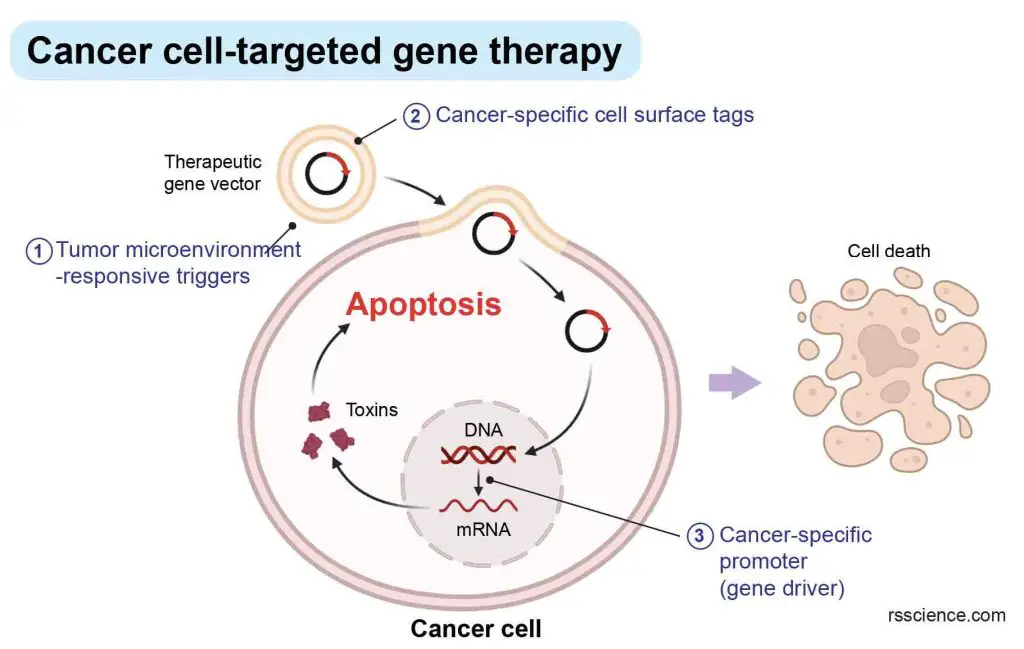
[In this image] The concept of cancer cell-targeted suicide gene therapy.
Oncolytic virotherapy
Oncolytic virotherapy involves using modified viruses to infect and destroy cancer cells selectively.
Immunotherapy
Immunotherapy involves modifying immune cells to recognize and attack cancer cells.
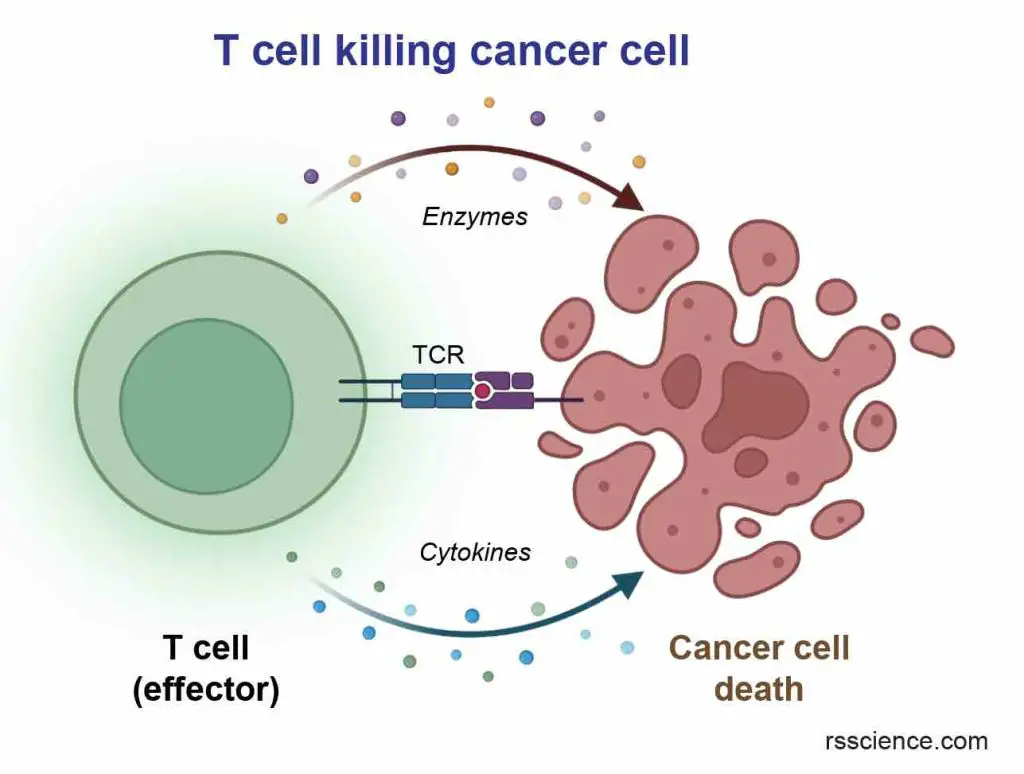
[In this image] The immune system has a natural ability to eliminate abnormal cells, such as virus-infected or cancerous cells. Unfortunately, cancer cells have evolved mechanisms to evade destruction by the immune system. The goal of immunotherapy is to boost or restore the ability of the immune system to recognize and attack cancers.
CAR-T (Chimeric Antigen Receptor T-cell) therapy is a type of immunotherapy that has recently shown great success in treating several types of blood cancer. It is an ex-vivo gene therapy that involves genetic modification of the patient’s T cells to produce a chimeric antigen receptor (CAR) on their surface. These engineered “CAR-T cells” gain boosted capability to destroy cancer cells.
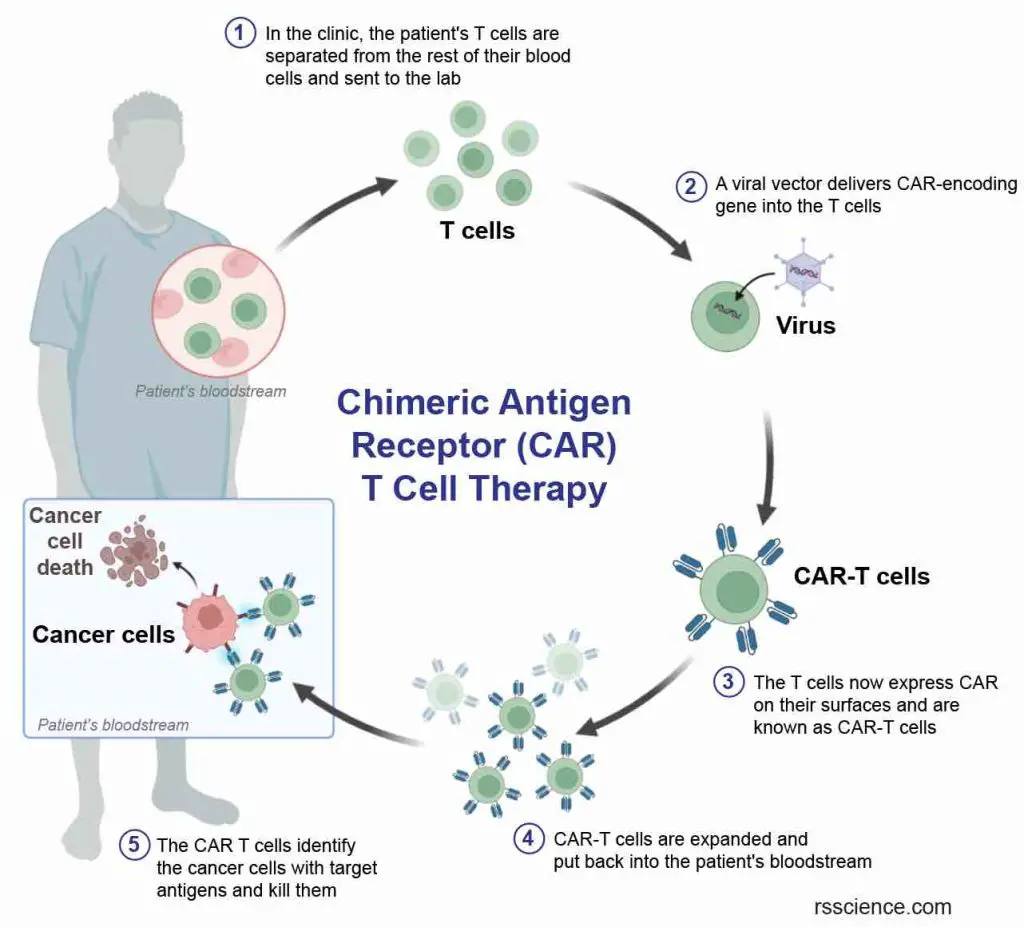
[In this image] The concept of Chimeric Antigen Receptor (CAR) T cell therapy.
CAR-T therapy involves several steps:
- Collection of T cells from the patient’s blood.
- The collected T cells are genetically modified in the lab to express chimeric antigen receptors (CARs) on their surface.
- These CARs are designed to recognize a specific antigen expressed in cancer cells.
- The modified T cells are expanded in the lab to produce a large number of CAR-T cells.
- The expanded CAR-T cells are infused back into the patient’s bloodstream.
The CAR receptor is designed to identify and attach to a specific protein on the cancer cell’s surface called an antigen. Once the CAR-T cells are transplanted back into the patient, they can identify and eliminate cancer cells that express the targeted antigen.
Although gene therapy for cancer has shown promising results in certain cases, it remains a developing field, and ongoing research is necessary to enhance its safety and efficacy. As of now, it is not a widely accessible treatment option for cancer.
Current CAR-T therapy has limited effectiveness against solid tumors, such as breast or brain cancer. However, researchers are exploring alternative gene therapy strategies to improve the treatment of solid cancers. Emerging technologies, like CAR-NK cells or TCR-T cells, may offer additional options to combat cancer in the future.
What are the potential risks of gene therapy?
Gene therapy has the potential to carry risks, which are dependent on various factors such as the type of therapy, delivery method, and the specific cells targeted. Some of the possible risks include the following:
- Negative immune system reaction
- Complications due to unintended targeting of cells (off-target effects)
- Adverse effects associated with the introduction of genetic materials
- Unexpected gene expression
To ensure the safety of gene therapy, clinical studies are conducted to evaluate its effectiveness and potential risks before regulatory bodies such as the Food and Drug Administration (FDA) approve it for human use. Additionally, ongoing research and monitoring are necessary to improve the safety and efficacy of gene therapy.
The field of gene therapy products is constantly evolving, and scientific advancements are being made at a rapid pace. The FDA is committed to supporting product developers and speeding up the development of groundbreaking treatments that have the potential to save lives by interacting with them and promptly reviewing their products.
A brief history of gene therapy
Below is a timeline featuring significant milestones in the history of gene therapy.
1866 – Gregor Mendel discovered the fundamentals of heredity and how genetic information is passed from parent to child.
1909 – Wihelm Johanssen proposed the term “gene”.
1953 – Rosalind Franklin, Francis Crick, and James Watson characterized the structure of DNA.
1961 – The first codon of the genetic code was decoded.
1968 – Stanfield Rogers and Peter Pfuderer demonstrated that virus-mediated gene transfer is possible.
1970 – The concept of gene therapy was first considered as a treatment for genetic diseases.
1972 – Recombinant DNA technology was established.
1980 – Martin Cline attempted gene therapy abroad without permission in 2 patients with beta-thalassemia, a rare inherited blood disorder, by transferring the beta-globin gene into their cells. This did not work because the cells did not replicate.
1989 – Steven Rosenberg conducted the first officially approved human gene therapy trial into five patients with advanced melanoma.
1990 – The first gene therapy clinical trial was conducted using new viral vector technology. 2 patients with severe combined immunodeficiency (SCID) received treatment.
1999 – The first person publicly identified as having died in a clinical trial for gene therapy. Jesse Gelsinger suffered from ornithine transcarbamylase deficiency, an X-linked genetic disease of the liver. Gelsinger was injected with an adenoviral vector carrying a corrected gene to test the safety of gene therapy. He died four days later. This temporarily slowed down the development of gene therapy.
2003 – China approved the first gene therapy product for clinical use.
2009 – First successful phase 3 gene therapy clinical trial in Europe.
2012 – The first gene therapy for the treatment of lipoprotein lipase deficiency (LPLD) was approved by European Medicines Agency.
2012 – Scientists developed a gene-editing technique called CRISPR/Cas9 that can modify specific DNA sequences.
2016 – The first gene addition therapy to treat adenosine deaminase severe combined immunodeficiency (ADA-SCID) was approved in Europe.
2017 – Nearly 2600 gene therapy clinical trials completed, ongoing, or approved worldwide.
2017 – The first gene therapy to treat patients with a rare form of inherited blindness was approved in the US.
2017 – The first CAR-T cell therapy for the treatment of B cell lymphoma was approved in the US.
2018 – The first clinical trial using CRISPR/Cas9 was initiated.
2022 – The first gene therapy to treat adults with Hemophilia B was approved. It costs $3.5 million per dose, which is the most expensive drug so far.
Summary
1. Gene therapy is a promising biomedical technology that aims to cure diseases by changing the patient’s DNA.
2. Gene therapy can be used to treat a wide range of genetic diseases, including inherited disorders and some types of cancer.
3. Gene therapy can be done by (1) Gene addition – introducing a healthy gene into the patient’s cells; (2) Gene silencing – turning off a disease-causing gene; (3) Gene editing – precisely correcting a mutated gene; and (4) Base editing – precisely re-writing a single DNA base pair to fix a mutation.
4. Gene therapy requires the usage of a “vector” to deliver therapeutic DNA into a patient’s cells. Common vectors include DNA plasmids, liposomes, nanoparticles, and genetically-modified viruses.
5. Viral vectors are commonly used for gene delivery because they are good at delivering genes into cells. Adenoviruses, adeno-associated viruses (AAVs), retroviruses, and lentiviruses are the most common viral vectors for gene therapy.
6. Gene therapy is still a relatively new field, and there are still many challenges and potential risks associated with this approach. Despite these challenges, gene therapy holds great promise for treating genetic diseases and improving patient outcomes in the future.
7. Gene therapy is currently being researched and tested in clinical trials around the world, and it is expected to become an increasingly important part of modern medicine in the years to come.