This article covers
What is cytoskeleton? A quick overview
The cytoskeleton is a network of filament proteins that extends throughout a cell. The cytoskeleton supports the cell, gives it shape, organizes and suspends the organelles within the cytoplasm, and has roles in molecule transport, cell division, cell signaling, and cell movement. Functionally, you can say the cytoskeleton network is equal to a cell’s muscle, bone, blood vessel, and nervous systems in combination.
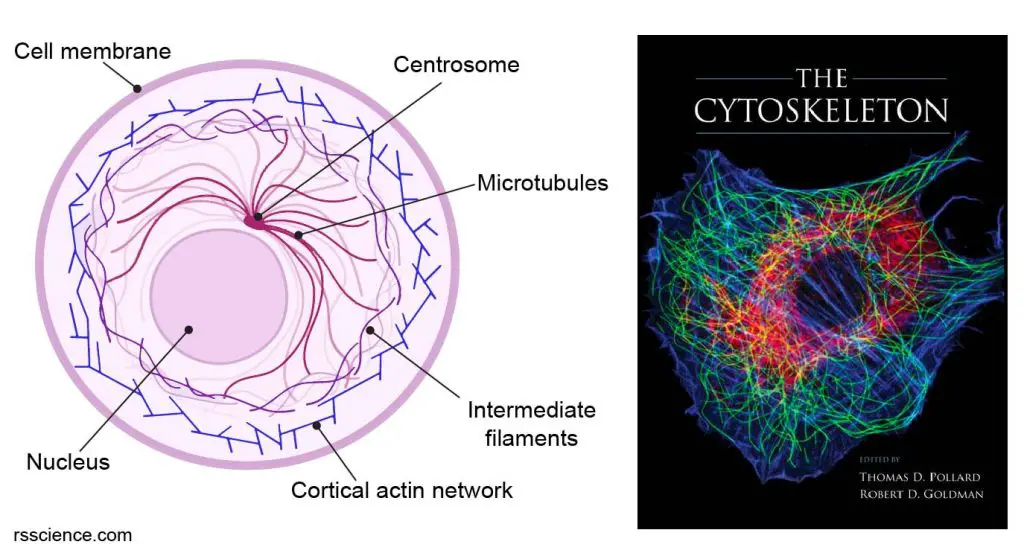
[In this figure] Left: A diagram of a cell showing the network of cytoskeleton consisting of three types of filament proteins – actin, intermediate filament, and microtubule.
Right: Immunofluorescence micrograph of a rat fibroblast stained simultaneously for microtubules (green), actin filaments (blue), and vimentin-type intermediate filaments (red). The image is a cover of “The Cytoskeleton”, published by Cold Spring Harbor Laboratory Press.
Cytoskeleton structure
What is the cytoskeleton made of
All cells have cytoskeleton, though the proteins that are made of vary between organisms. The eukaryotic cytoskeleton consists of three types of filaments: actin, microtubule, and intermediate filaments. In contrast, The prokaryotic cells also have their cytoskeleton, but made of different protein units.
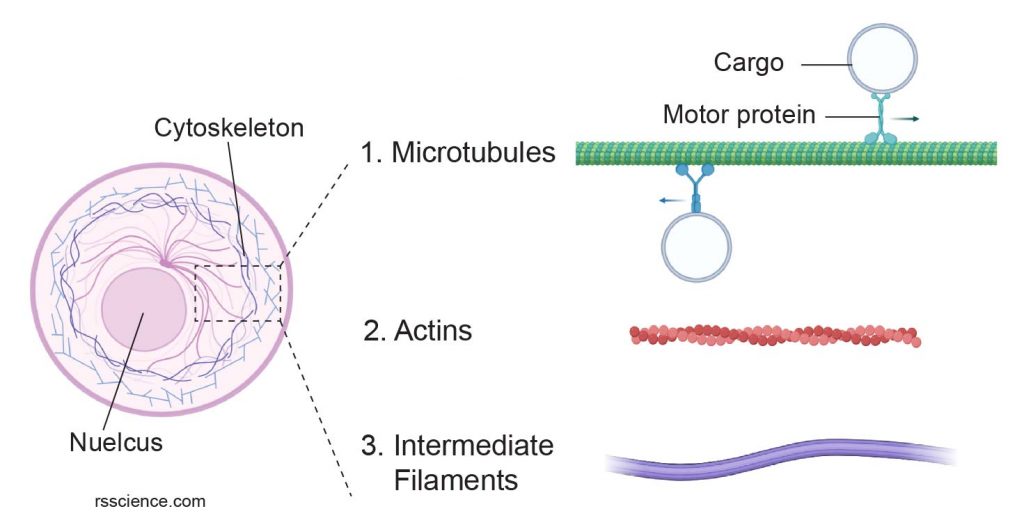
[In this figure] Cytoskeleton consists of three types of filament proteins: microtubules, actins, and intermediate filaments.
The image was created with BioRender.com
Actin filaments
Actin filaments (also called microfilaments) compose of the protein “actin”. Actins exist in two forms: G-actin (monomeric globular actin) and F-actin (polymeric fibrous actin). F-actins (or actin filaments) are two strands of G-actins wrapped in a spiral. An actin filament is about 7 nanometers thick, making them the thinnest filaments in the cytoskeleton.
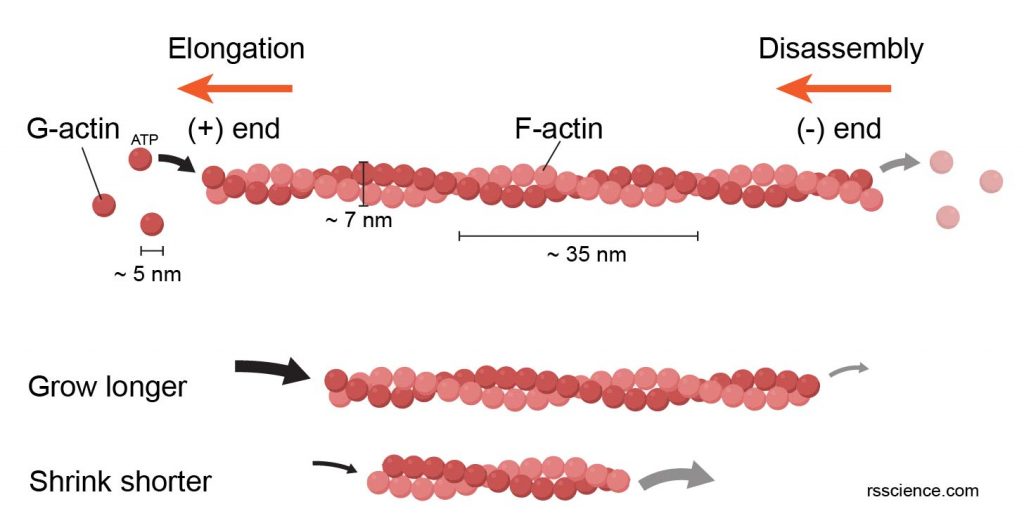
[In this figure] The polymerization of G-actin units into a F-actin filament.
Actin filaments are dynamic structures. New G-actins join the chains (requiring ATP as energy) at the (+) end, making the filaments grow toward the direction where actins are added. The actin disassembles from the (-) end, resulting in the shortening at the (-) end. If the polymerization rate at the (+) end is higher than the depolymerization rate at the (-) end, the filament can grow longer; and vice versa. This dynamic cycle allows the cell to allocate its actin networks toward the moving direction.
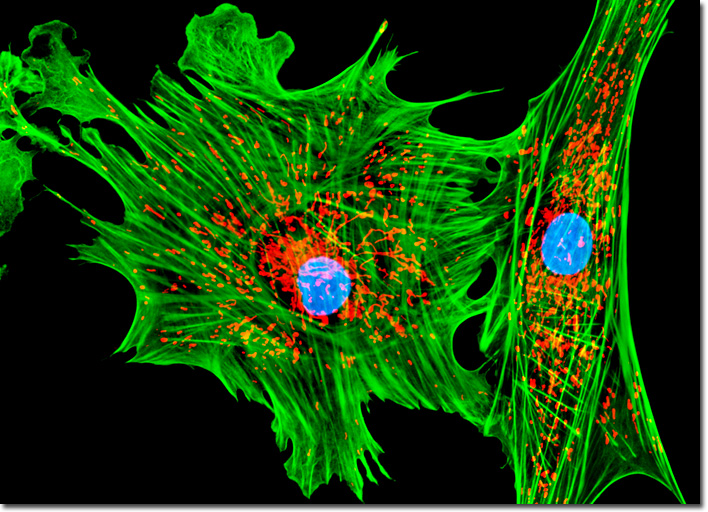
[In this figure] Fluorescent image of actin filaments (green), mitochondria (red), and nuclei (blue) in tahr ovary epithelial cells.
Photo source: Michael W. Davidson and The Florida State University
Actin filaments have many functions. A large portion of actin filaments concentrate near the cell membrane and form a cortical layer of actin networks. They control cell motility and cell shapes. They are also involved in cytoplasmic streaming and cell division. In muscle cells, actin filaments are one of the main components of muscular contractile elements which allow these cells to contract. We will discuss the functions of cytoskeletons later in detail.
Intermediate Filaments
Intermediate filaments (about 8-12 nm wide) got the name because their size is in between the size of actin filaments and microtubules. Intermediate filaments are made of a family of related proteins sharing common structural features. Examples are keratin (found in hair and nails, and also in animals with scales, horns, or hooves), vimentin, desmin, and lamin. Except for lamin, other intermediate filaments are found in the cytoplasm. Lamins are also found in the nucleus and help support the nuclear envelope that encloses the nucleus.
Unlike actin filaments, which grow and disassemble quickly, intermediate filaments are more permanent and play an essential structural role in the cell. They maintain the cell shape, bear tension, and anchor the nucleus and other organelles in place. Different cell types (i.e., epithelial cells vs. fibroblasts) have their unique compositions of intermediate filaments. This unique information could be used by scientists to learn the origins of cell types.
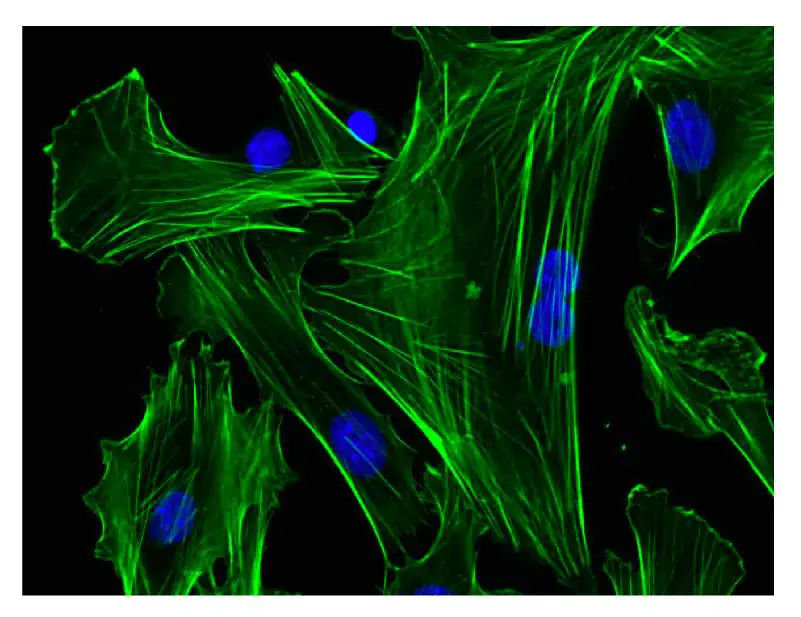
[In this figure] Fluorescent image of vimentin, an intermediate filament protein (green), in human cells. The nuclei were stained in blue color.
Microtubules
Microtubules are the thickest fibers of the cytoskeleton at about 23-27 nm. They are hollow tubes made of two globular proteins – alpha (α) and beta (β) tubulin. The polymerization of α- and β-tubulin dimers (pairs) into protofilaments that can then associate laterally to form the hollow tube. The most common form of microtubules consists of 13 protofilaments in the tubular arrangement.
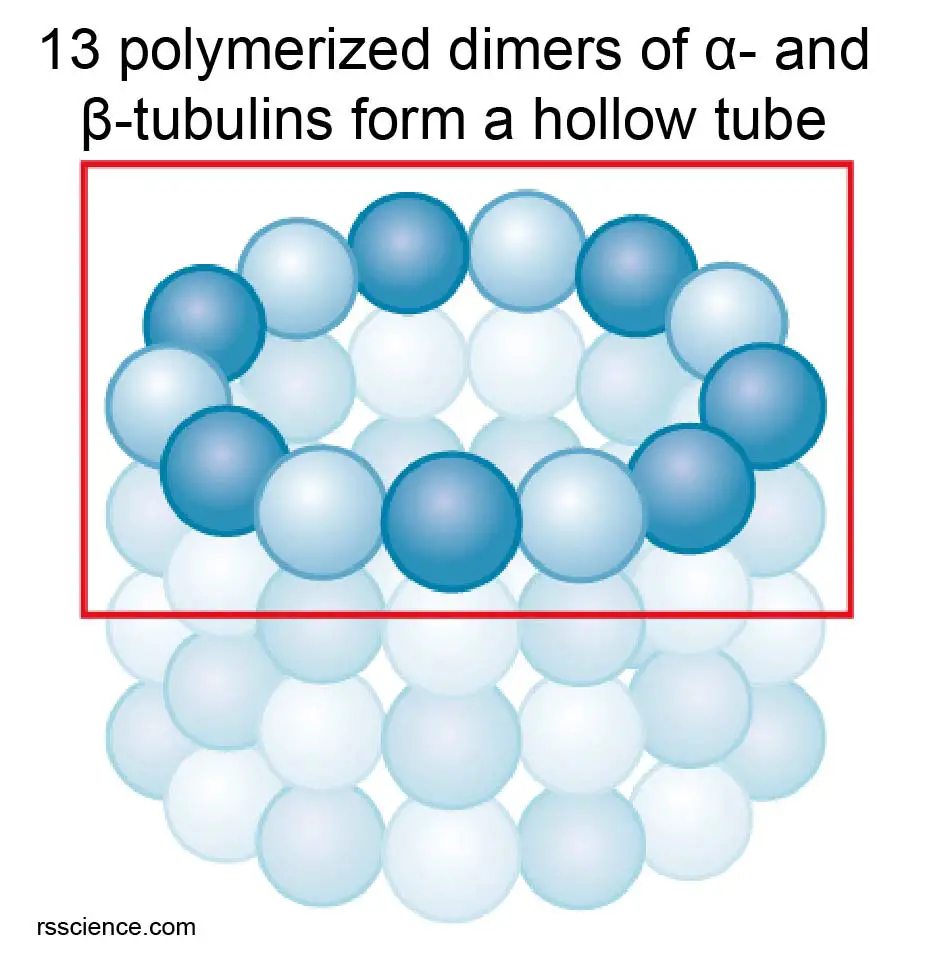
[In this figure] The hollow tube structure of microtubules is formed by an array of α‑tubulin/-β‑tubulin dimers.
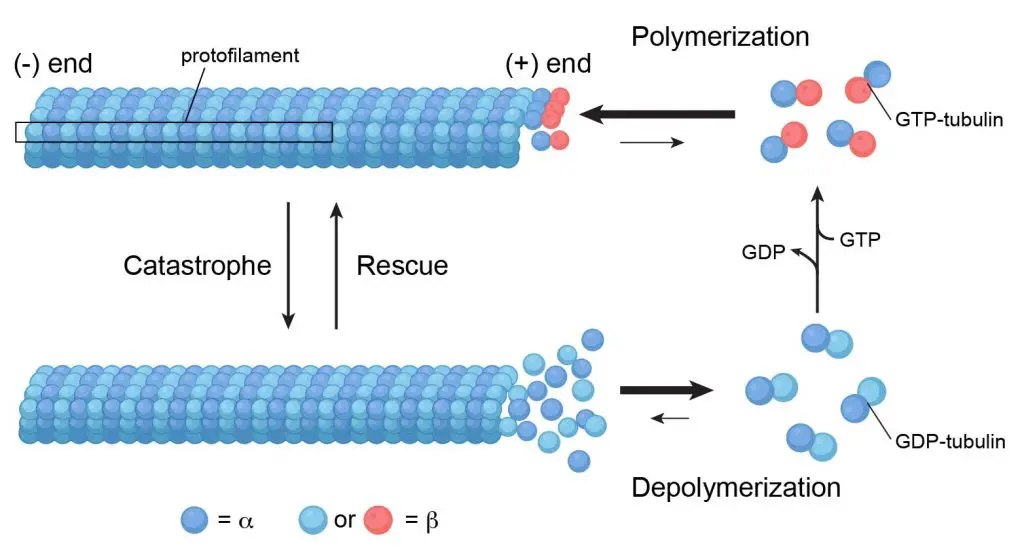
[In this figure] Microtubules are assembled from α‑tubulin and β‑tubulin. Soluble α‑tubulin/-β‑tubulin dimers polymerize into polar microtubules in the presence of GTP (energetic molecules similar to ATP).
Microtubules are also a group of versatile cytoskeleton filaments. Most of the microtubules in an animal cell come from a cell organelle called centrosome, which is a microtubule organizing center (MTOC). The centrosome is found near the middle of the cell, and microtubules radiate outward from it. Microtubules are anchored at the MTOC by their minus (-) ends, while their plus (+) ends continue to grow into the cell periphery.

[In this figure] The organization of microtubule in different cell types.
The microtubule organization varies according to the cell types and cell cycle. It determines the internal organization of organelles and vesicular trafficking that benefit the cell functions.
Photo source: Atlas of plant and animal histology
During cell division, microtubules form the mitotic spindle, which separates sister chromatids equally apart so that each copy can go to each daughter cell. Microtubules also involved in transporting molecules within the cells and the formation of cellular structures, such as flagella and cilia.
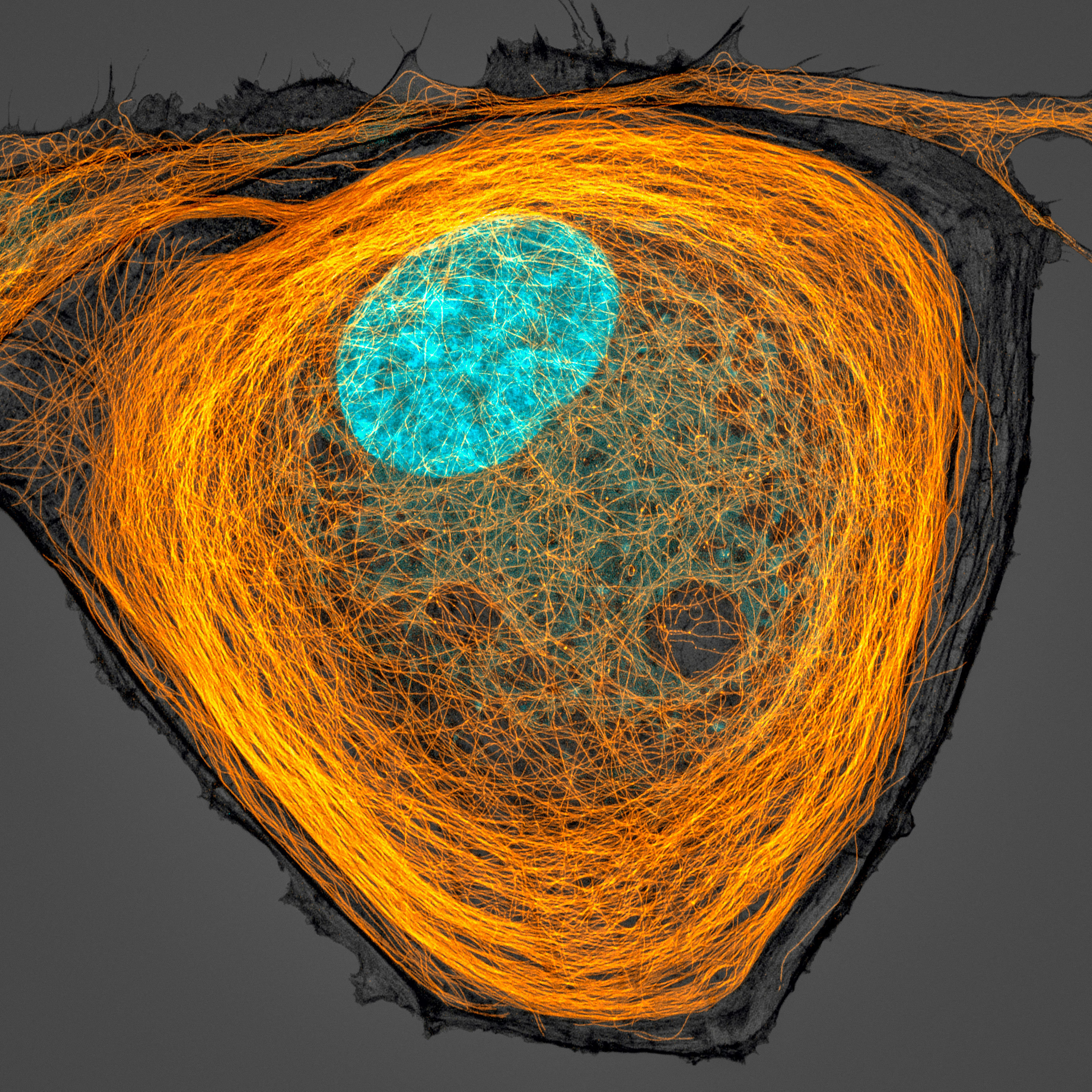
[In this figure] Fluorescence image of microtubule (orange), and the nucleus (cyan) inside a cell.
Microtubule is one type of cytoskeleton inside the cells, and it shapes cell’s morphology. Magnification, 63x.
Photo credit: Jason Kirk, 2020 photomicrograph competition.

[In this figure] Transmission electron microscopy (TEM) image showing parallelly-oriented microtubules inside a dendrite of a neuron.
Photo source: Atlas of plant and animal histology
Why the cytoskeleton is important – the cytoskeleton function
Cell shape and integrity
The primary function of the cytoskeleton is to give the cell its shape and mechanical resistance to deformation. This is especially important for cells without cell walls, such as animal cells, which do not get their fixed shape from a thick outer layer.
The filaments of the cytoskeleton anchor to the inner face of the cell membrane by binding to adhesion proteins like integrins and cadherins. These adhesion proteins, on the extracellular side, interact with neighbor cells and extracellular matrix (ECM).
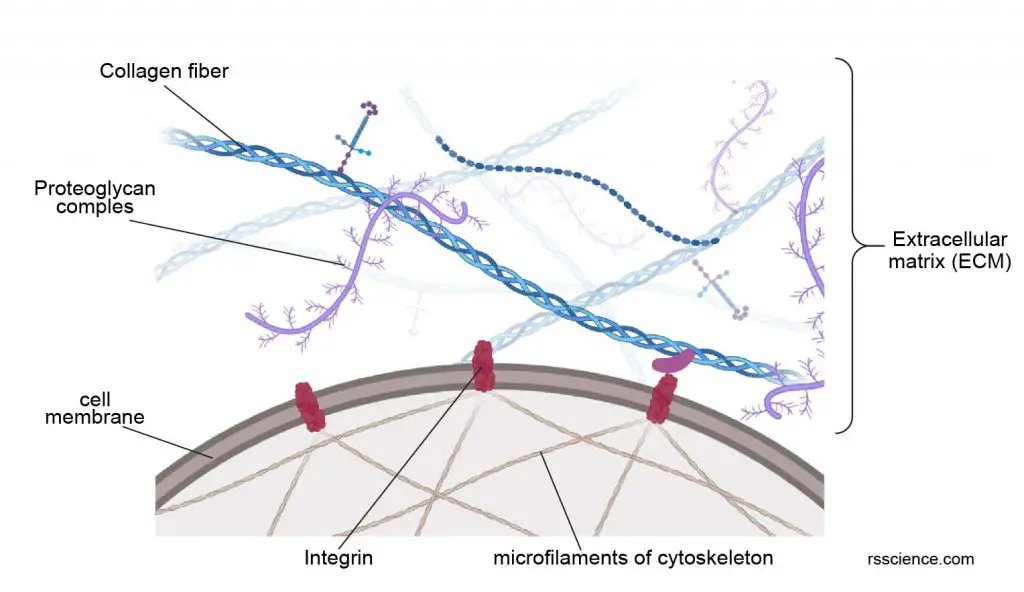
[In this figure] Extracellular matrix (ECM) is a three-dimensional network consisting of several types of filament proteins secreted by the cells. The most well-known example is collagen fibers. ECM provides additional protection for cells. Cells adhere to the ECM bed through the anchoring proteins called integrins. The parts of integrins inside the cytoplasm attach to the cytoskeleton network. Overall, this provides integrity not only for individual cells but also for tissue as a whole.
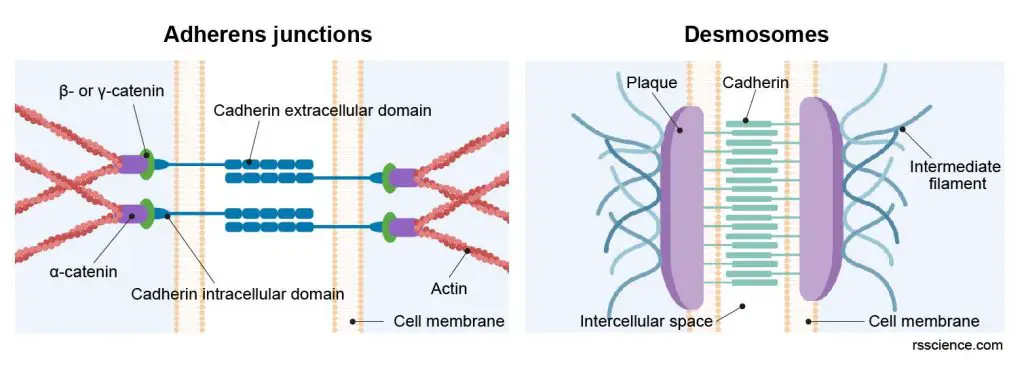
[In this figure] Two examples of junctional complexes which bring two neighboring cells together.
Adherens junctions link to actin filaments whereas desmosomes connect intermediate filaments from two adjust heart muscle cells. The linked cytoskeleton networks enable the transmission of contractile force from one cell to another.
Cell movement
The cytoskeleton network reaches every inch inside the cells. Therefore, once a portion of the cytoskeleton contracts or extends, it deforms the cells and allows cells to change their shapes and movement. This is especially important in single-celled organisms like amoebas.
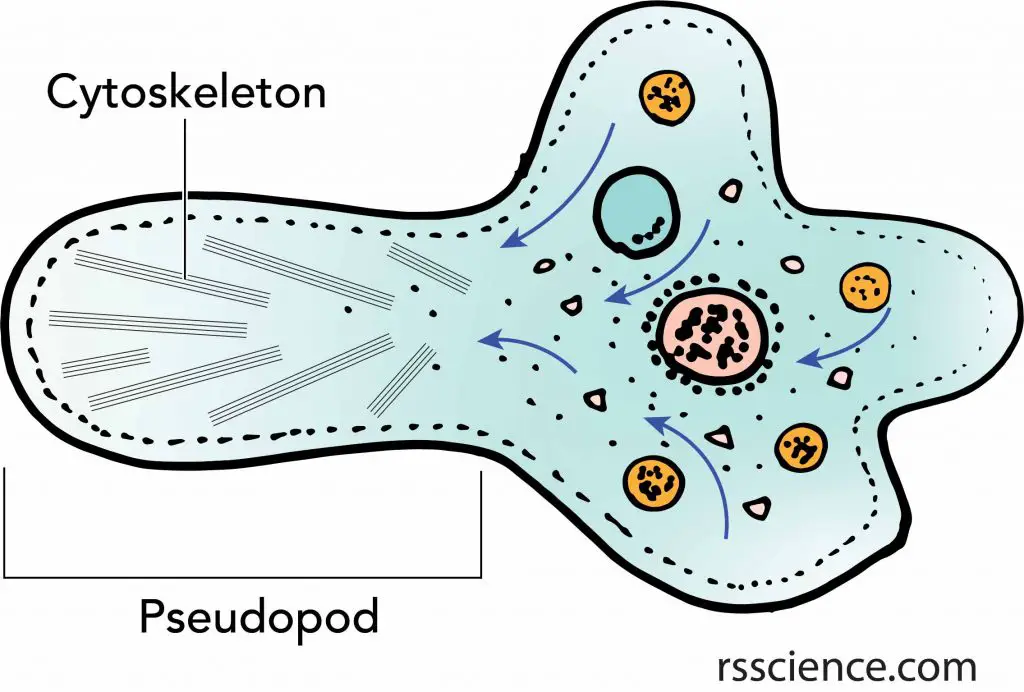
[In this figure] Amoeba’s pseudopod is an excellent example of how cells use the cytoskeleton to move.
The change of cell shapes by rearrangement of cytoskeleton allows a cell to “engulf” objects from the outside world. Examples are the “phagocytosis” of amoebas or “endocytosis” of other cells.
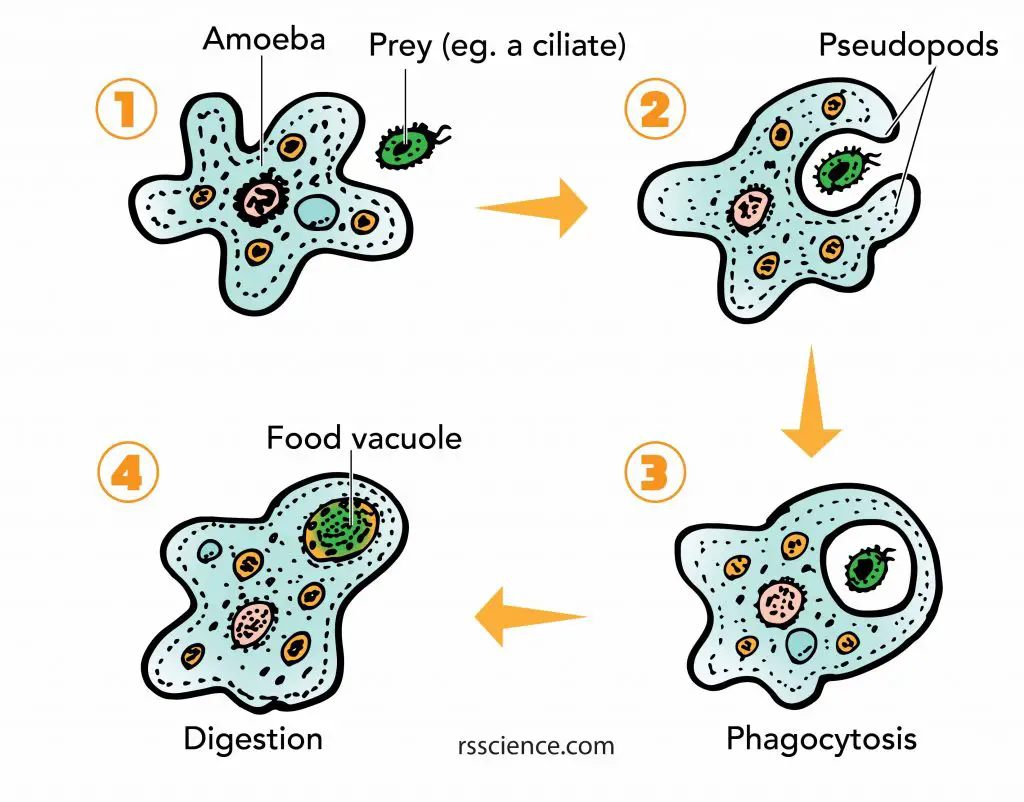
[In this figure] Amoeba phagocytosis.
The pseudopods first surround and bring the food particle close to the Amoeba. Then a part of the cell membrane opens to allow the particle to move into the cell and into a food vacuole where it is digested by enzymes.
Structure for flagella and cilia
Cytoskeleton also supports special cellular structures that allow cells to move. Microtubules form the core structures of flagella (singular: flagellum), which are “tails” that propel a cell forward. Microtubules are also found inside the cilia (singular: cilium), which are hair-like small protrusions. These cilia are in constant motion and help single-celled microorganisms, such as paramecium, move around.
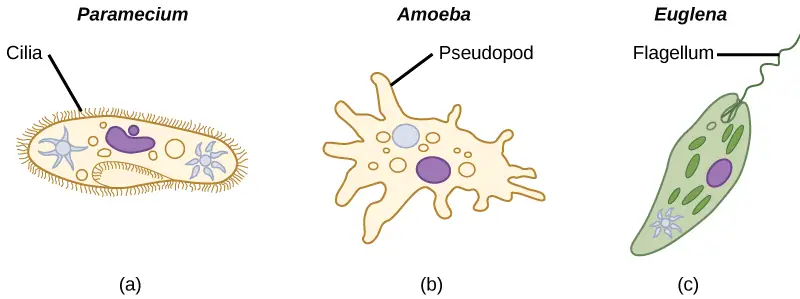
[In this figure] Three ways of locomotion for protozoa.
Cilia – coordinately beat to swim. Pseudopod – crawl on the surface by changing the cell shape. Flagellum – swim by rotating like a propeller.
Photo credit: Lumen.
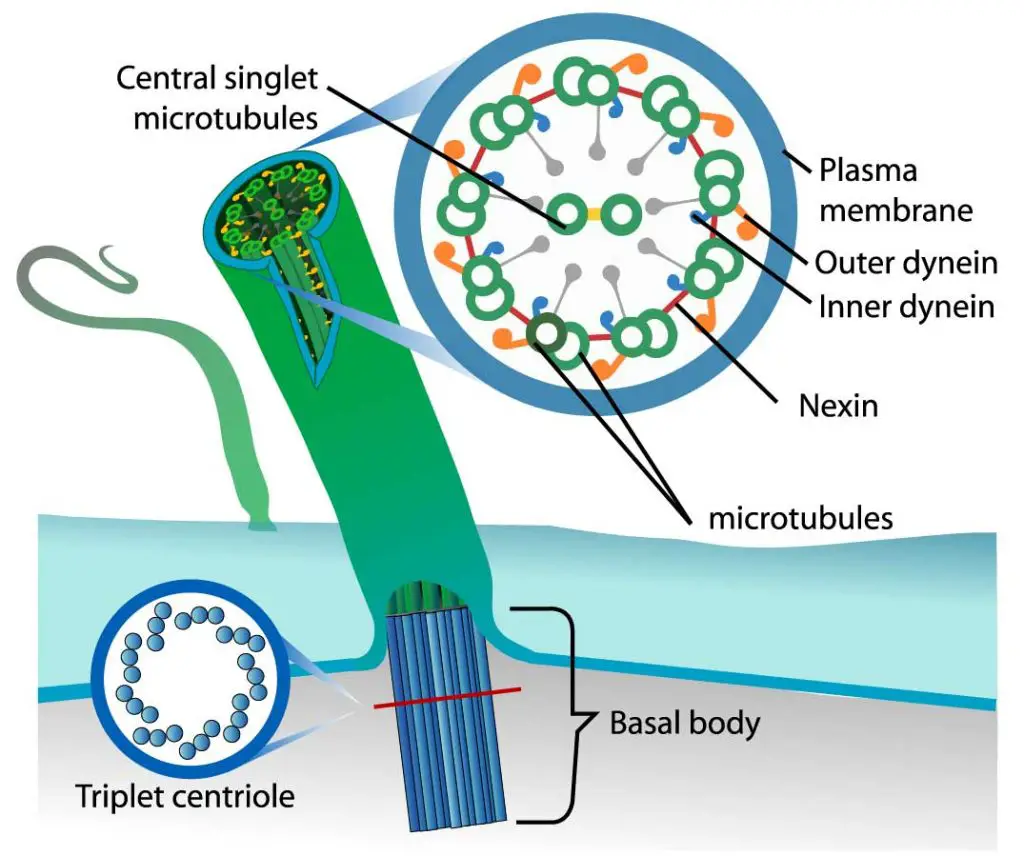
[In this figure] The organization of cilium.
Each cilium contains nine pairs of microtubules forming the outside of a ring and two central microtubules. This structure is known as an axoneme. Microtubules are held together by cross-linking proteins. There are motor proteins, called dynein, setting across each paired microtubule fiber.
Photo credit: LadyofHats on wiki.
The microtubules function in a similar way in both flagella and cilia. The motor proteins (dynein) use ATP as energy to crawl along the microtubules. When dynein proteins move upward on one side but down on the other side, the cilium bends. The repeat of bending-relaxing cycles makes cilia act like oars, beating back and forth to create movement.
Transportation inside the cells
The cytoskeleton is literally a highway system inside the cells. There is a group of “motor proteins” that can carry cargos while walking along the cytoskeleton, which is just like many small trucks driving on an intracellular transportation system. A variety of intracellular cargos, including proteins, RNAs, vesicles, and even entire organelles, can move around in a cell by sitting on these motor proteins.
[In this video] An animation showing that the motor protein (Kinesin) carries cargo and walks on the cytoskeleton of the microtubule.
How do motor proteins work?
Motor proteins have two “hands” and two “feet”. The hands and feet are both “sticky” – like post-it-notes. Just as a human can walk by placing one foot in front of the other, the motor proteins “walk” by swinging one foot in front of the other. Motor proteins walk along with the filament proteins of cytoskeletons, much like a trapeze artist walking on a high wire. At the same time, motor proteins can carry cargos using their hands. The sticky patches on the “hands” prevent the cargo from falling off as the motor proteins set off on their journey.
Motor proteins use adenosine triphosphate (ATP) as the energy currency to move. Each step uses one molecule of energy. The motor proteins need 125,000 steps to move 1mm along the cytoskeletons – that is a lot of energy!
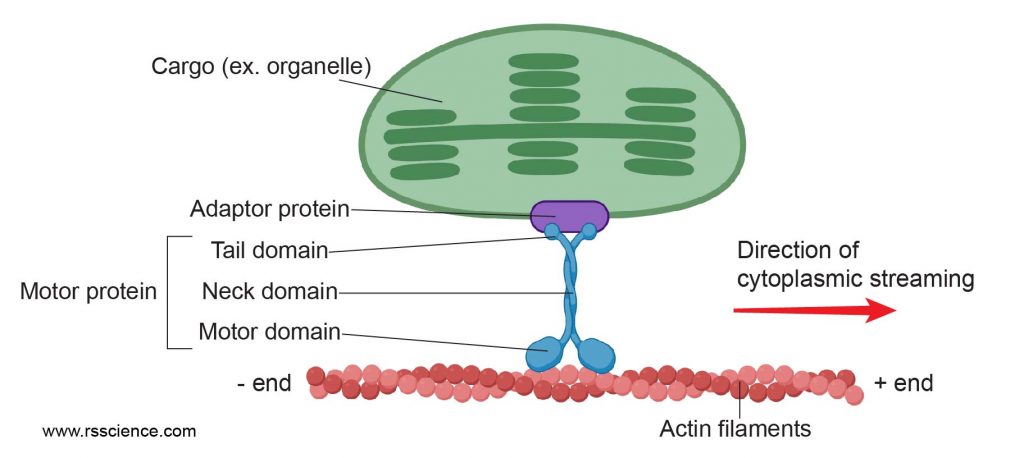
[In this figure] Motor protein walks on a molecular catwalk made of cytoskeletons (here, actin filaments) to deliver cargos (for example, a chloroplast). This movement also generates a flow of cytosol, resulting in cytoplasmic streaming.
Cytoplasm streaming
Cytoplasmic streaming plays an important role in plant cells and large single-celled protists because it can enhance the exchange of vital molecules inside these cells.
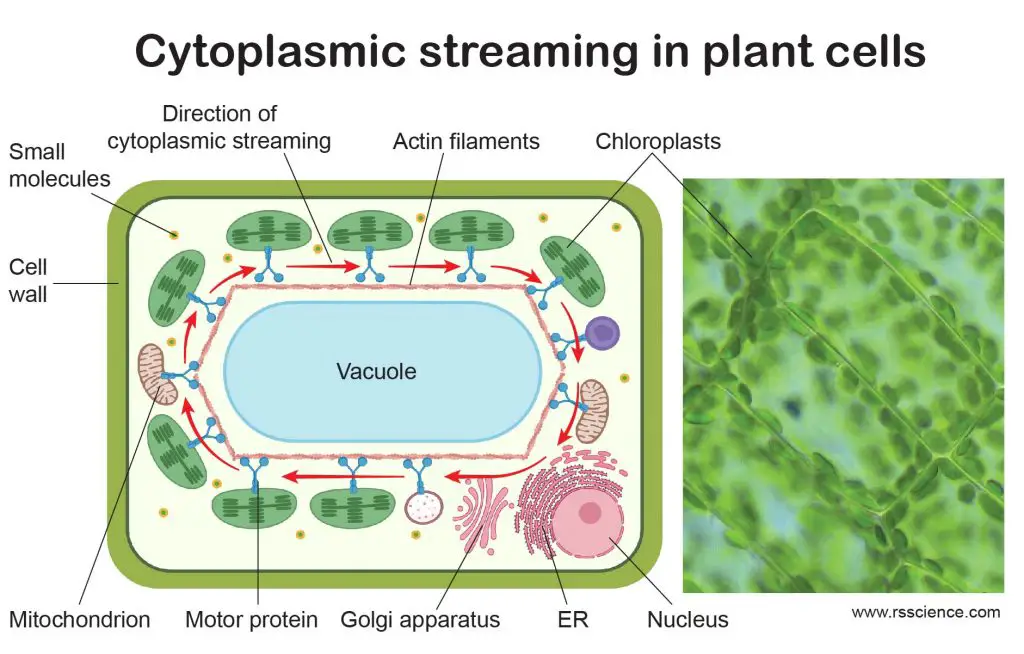
[In this figure] Cytoplasmic streaming in plant cells.
Cytoplasmic streaming circulates the chloroplasts around the central vacuoles in plant cells. This optimizes the exposure of light on every single chloroplast evenly, which can maximize the efficiency of photosynthesis. The right image is the actual cytoplasmic streaming of chloroplasts in Elodea cells.
Created with BioRender.com
Cytoplasmic streaming is mediated by “motor” proteins “walking” along the cytoskeleton. When motor proteins drag organelles to move, the motion also creates a current surrounding the cytosol. If many motor proteins move along one direction (for example, move clockwise around the central vacuole in a plant cell), many of these small flows join and become powerful cytoplasmic streaming. Dragging the endoplasmic reticulum (ER) is the most efficient way to generate cytoplasmic streaming. This is because that ER is a web-like structure with a significant surface area interacting with the cytosol. You can imagine that there are many fishing boats trawling together inside a small cell.
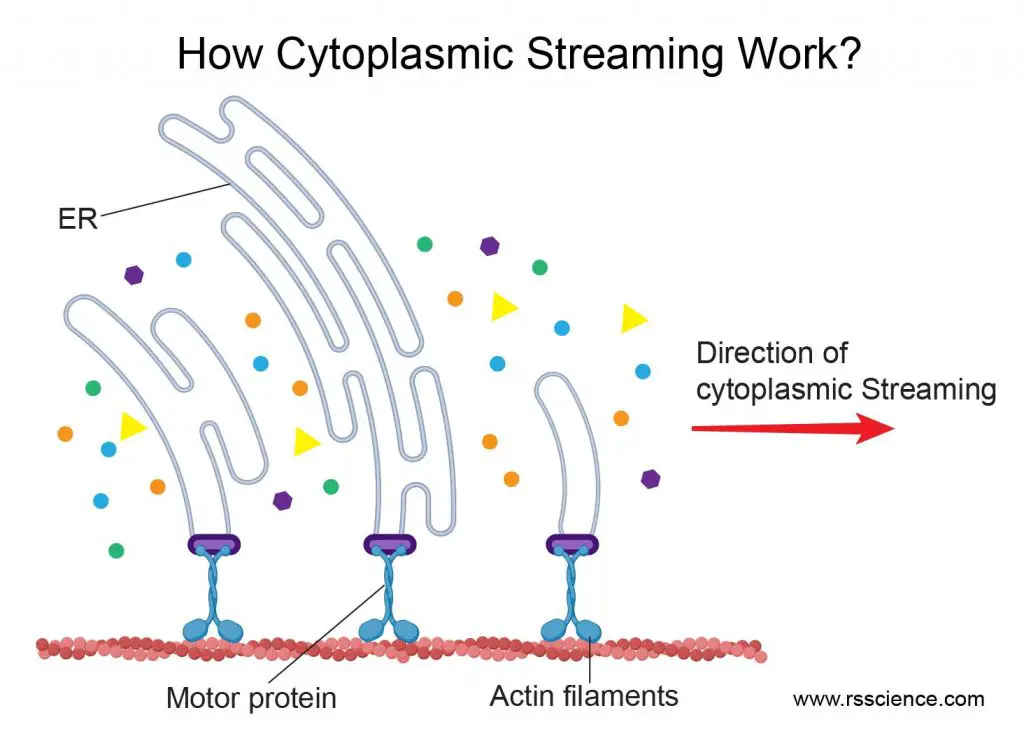
[In this figure] Motor proteins dragging the movement of ER webs can efficiently generate cytoplasmic streaming in the cell.
Created with BioRender.com
Cell division
Centrosomes are organelles that serve as the main microtubule organizing center (MTOC) during mitosis. Each cell has two centrosomes. When mitosis starts, two centrosomes move toward the opposite positions of the cells and form a mitotic spindle. The microtubules extend from the centrosome and attach to the centromeres of sister chromatids. Then, both centromeres retrieve their microtubule at the same time. The contraction force separates the sister chromatids apart from the attachment points of the centromere; then moves toward the opposite direction. Once all sister chromatids are equally separated into two new nuclei, the cytoplasm and cell membrane will also divide into two new cells.
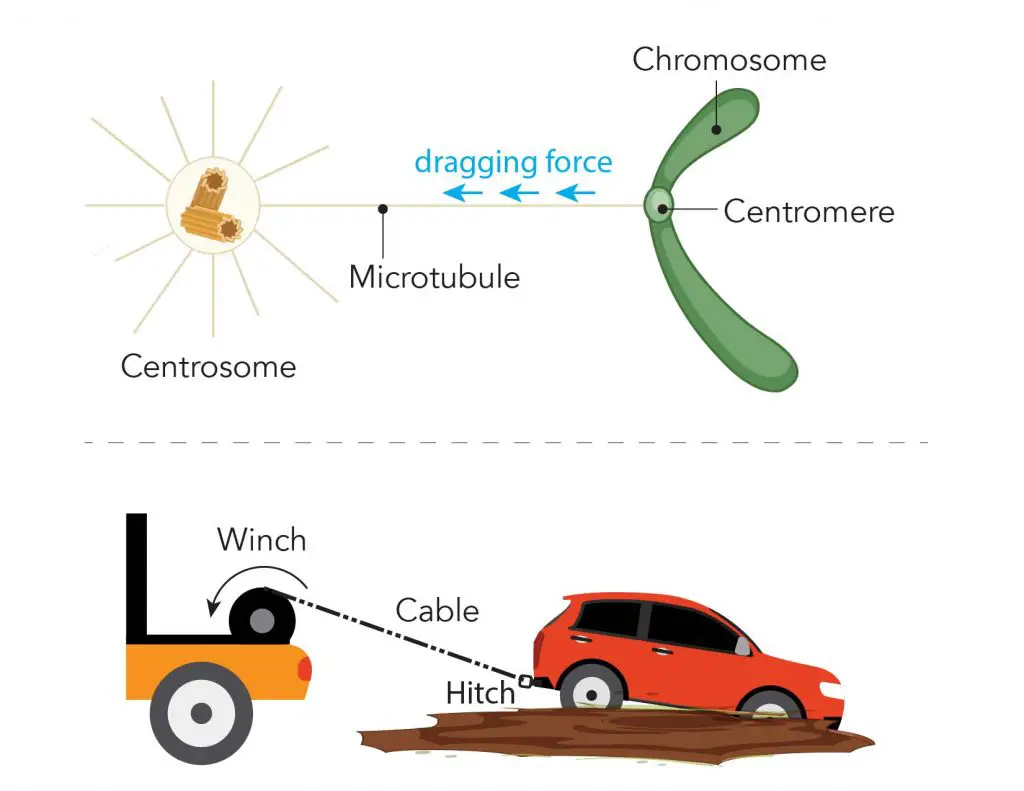
[In this figure] Illustration of microtubule, centromere, and centrosome during mitosis.
During mitosis, one end of the microtubule attached to the centromere of each chromosome, and the other ends are controlled by a special organelle, called “centrosomes”. You can imagine that the centrosome is like the winch pulling the sister chromatids (the car) apart by dragging the microtubule (the cable) anchored to the centromere (the tow hitch).
Muscle contraction
Muscle cells are equipped with bundles of myofibrils that contain myofilaments. The myofilaments are organized cytoskeleton fibers consisting of actins and myosins.
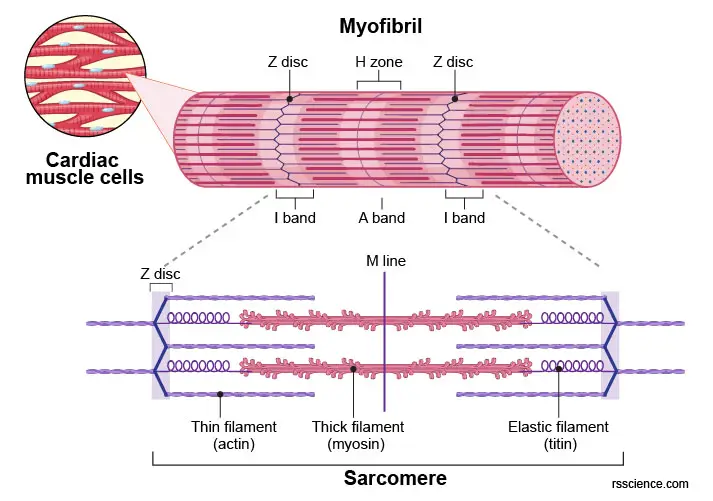
[In this figure] Labeled diagram of myofibril showing the organization of thin (actin) filaments and thick (myosin) filaments.
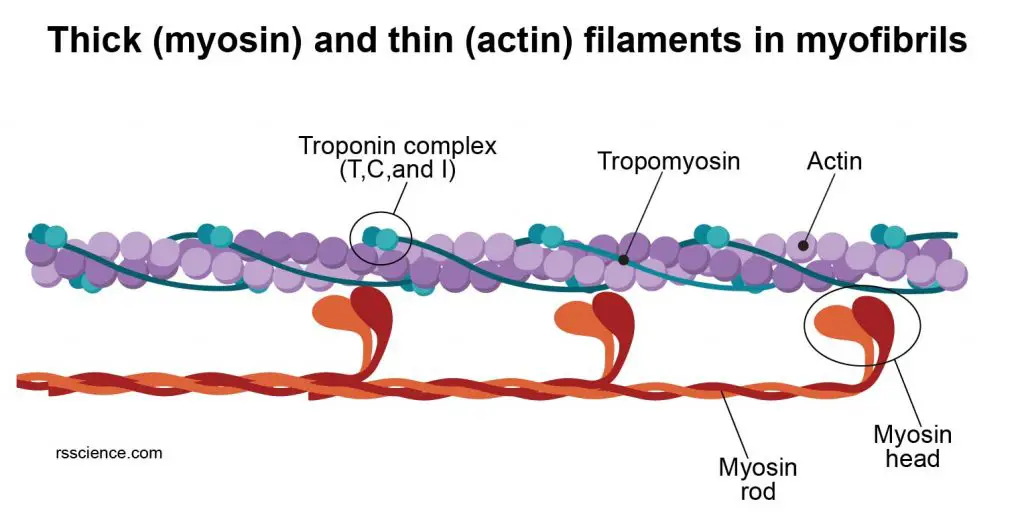
[In this figure] The heads of myosins interact with the actin filaments.
To generate muscular contraction, the myosin heads bind to actin filaments, allowing myosin to function as a motor that drives filament sliding. The actin filaments slide past the myosin filaments toward the middle of the sarcomere. The result is shortening of the sarcomere without any change in filament length.
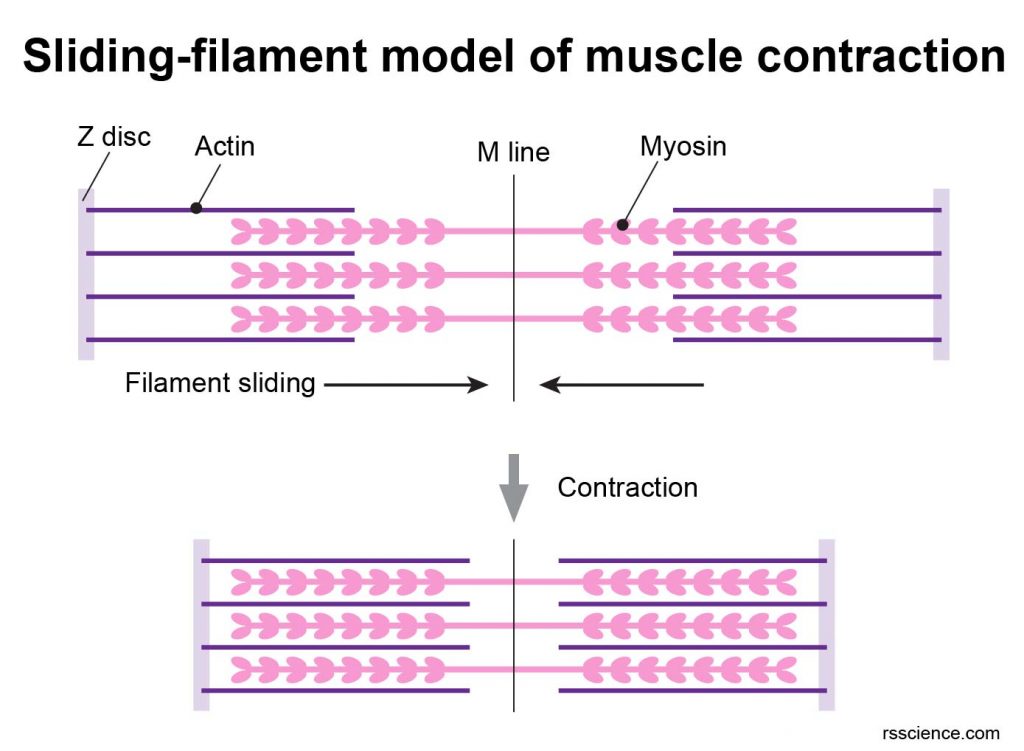
[In this figure] Sliding-filament model of muscle contraction.
Bacteria that hijack the cell’s cytoskeleton to move!
Many bacteria can infect and enter a host cell. Among them, Listeria Monocytogenes are definitely the unique one: these bacteria can hijack the cell’s actin network to help them move around!
[In this video] Listeria Infection in a cell.
Dark rod-shaped objects are Listeria bacteria. You can see them move rapidly inside the cell by riding on the pointed end of actin filaments.
Q&A:
Do bacteria have cytoskeleton?
All cells have cytoskeletons. Usually, when we mention the cytoskeleton, we referred to eukaryotic cells’ cytoskeleton. Prokaryotic cells (including bacteria and archaea) were originally thought not to have a cytoskeleton. Until the early 1990s, scientists discovered unique cytoskeleton-like proteins in bacteria. For example, FtsZ is a bacterial protein that functions like tubulins and helps bacterial cell division.
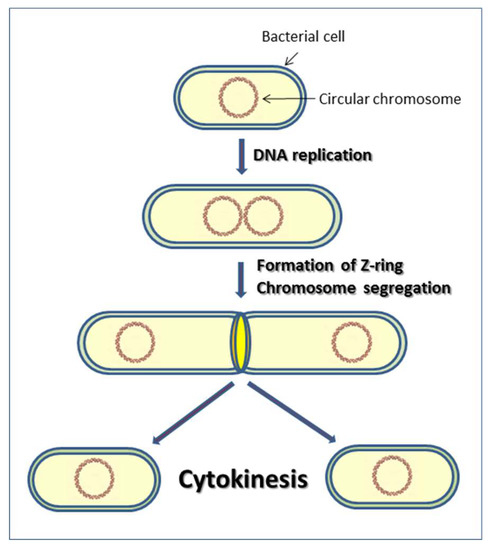
[In this figure] At the end of bacterial cell division, FtsZ proteins assemble at the middle of the cell in a ring-like structure. This ring shrinks to force the cell wall to divide into two new cells.
Photo source: MDPI
How to view the cytoskeleton under a microscope?
The protein filaments of the cytoskeleton are too thin to be seen under a regular light microscope. Fluorescent microscopes with immunofluorescent staining are the standard method to observe and study cytoskeleton components. Specific fluorescent-labeled antibodies recognized cytoskeletal protein (such as actin and tubulin), resulting in a beautiful visualization of cytoskeletal network.
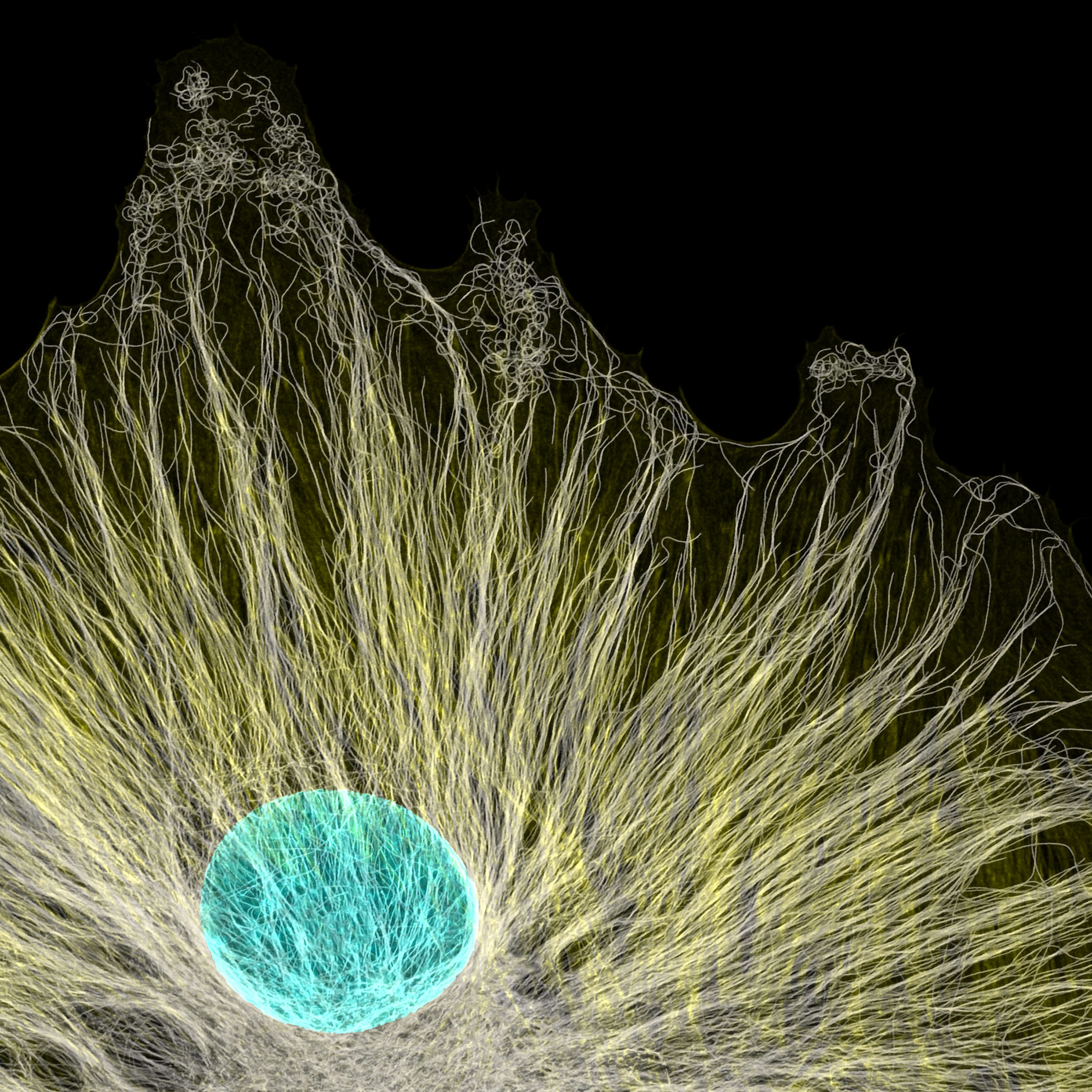
[In this figure] Fluorescence image of microtubule (yellow) and the nucleus (cyan) inside a cell.
Microtubules radiated from a tissue cell culture. Notice that the microtubules extend to the very end of the cell membrane. Magnification, 63x.
Photo credit: Jason Kirk, 2020 photomicrograph competition.
Electron microscopy (EM) is another important tool to study the cytoskeleton, especially the structures and sizes of protein filaments in detail.

[In this figure] Electron micrographs of microtubules, actin filaments, and intermediate filaments.
Photo source: Migrations.
Summary
- Cytoskeleton is the cells’ skeleton system. Its network reaches every inch inside the cells.
- Cytoskeleton is a dynamic network built by interlinking protein filaments. It is composed of three main components, actin filaments (microfilaments), intermediate filaments, and microtubules.
- Once a portion of the cytoskeleton contracts or extends, it deforms the cells and allows cells to change their shapes and movement.
- Cytoskeleton also serves as a highway system inside the cytosol. Motor proteins can carry cargos while walking along the cytoskeleton. A variety of intracellular cargos, including proteins, RNAs, vesicles, and even entire organelles, can move around inside a cell by this intracellular transportation system.
References
“The Cytoskeleton” Cold Spring Harbor Laboratory Press
“Basic Concepts: The Cytoskeleton”
What is cytoskeleton?
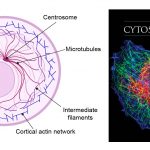
The cytoskeleton is a network of filament proteins that extends throughout a cell. The cytoskeleton supports the cell, gives it shape, organizes and suspends the organelles within the cytoplasm, and has roles in molecule transport, cell division, cell signaling, and cell movement. Functionally, you can say the cytoskeleton network is equal to a cell’s muscle, bone, blood vessel, and nervous systems in combination.